DNA Replication
Sakshi Education
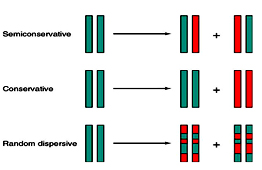
DNA replication is the process of duplication of genetic material or genome. In case of viruses and plasmids, DNA replication is a process of synthesizing multiple copies. The mechanism of DNA replication was proposed by Watson and Crick to be a semi conservative model. But later two other models were also put forward as Conservative model and Dispersive model. Semi conservative model as well accepted in which after the enzymatic synthesis of DNA, the resultant DNA consists of one polynucleotide chain from parent and newly synthesized complementary strand. The experimental proof for Semi conservative model was given by Messelson and Stahl.
Messelson and Stahl Experiment:
The experiment carried out by Meselson and Stahl involved growing a culture of Escherichia coli in a medium containing 15NH4Cl (ammonium chloride labelled with the heavy isotope of nitrogen). The labelled bacterial culture was transferred to a14N containing media and density of their DNA was monitored as a function of bacteria growth by" equilibrium density gradient ultracentrifugation". samples were taken after 20 minutes (one cell division) and 40 minutes (two cell divisions). DNA was extracted from each sample and the molecules were analyzed by density gradient centrifugation. After 20 minutes the entire DNA contained similar amounts of 14N and 15N, but after 40 minutes two bands were seen, one corresponding to hybrid 14N-15N-DNA, and the other corresponding to DNA molecules made entirely of 14N. The predicted outcome of the experiment is shown for each of the three possible modes of DNA replication.
The banding pattern seen after 20 minutes enables conservative replication to be discounted because this scheme predicts that after one round of replication there will be two different types of double helix, one containing just 15N and the other containing just 14N. The single 14N-15N-DNA band that was actually seen after 20 minutes is compatible with both dispersive and semi-conservative replication, but the two bands seen after 40 minutes are consistent only with semi-conservative replication. Dispersive replication continues to give hybrid 14N-15N molecules after two rounds of replication, whereas the granddaughter molecules produced at this stage by semi-conservative replication include two that are made entirely of 14N-DNA.
Semi discontinuous mode of DNA replication:
If the DNA replication mechanism were to be in a continuous mode, the DNA replication machinery should synthesize DNA is both 5'->3' and 3'->5' direction. But the DNA polymerase of all natural replicating machines can makes DNA is only direction 5'->3'
Reiji Okazaki concluded that DNA polymerase could theoretically make one strand continuously and other discontinuously. This discontinuity in DNA synthesis on one of the strand is due to its opposite orientation to the direction in which the replication fork is moving. So this strand is synthesized as fragments one behind the other. Okazaki and his colleagues performed experiments to prove the existence of such DNA fragments during replication. They labelled T4 phage DNA in short pulses and observed DNA pieces at very initial time points of DNA, when these investigators used T4 ligase mutants the pieces remained larger.
Priming of DNA synthesis:
Initiation of DNA synthesis requires a RNA primer, which is further extended by DNA polymerase at its 3' end adding nucleotides. Experimental evidence supporting RNA priming was given when replication of M13 phage in E.coli extracts was inhibited on addition of "rifampicin", an antibiotic that inhibits E.coli "RNAP".
Tuneki Okazaki (wife of Reiji Okazaki) and his co-workers purified 10-12 nucleotides RNA primers. They used mutant bacteria which lack RNAse H or nuclease activity of DNA pol I or both. To label intact primer they used the capping enzyme, gunaylyl transferase and a-32P-GTP to label 5' end of RNAs. After labelling they removed DNA portion with DNAse and subjected to gel electrophoresis which yielded primers of 11-12 nucleotides long in all lanes.
Bi-directional DNA replication:
Most eukaryotic and bacterial DNA replicates Bi-directionally. With two replication forks moving opposite to each other the DNA replicates.
Elizabeth and wake performed experiment on B.subtilis, as they have grown these cells initially in a medium with weakly radioactive DNA precursor followed by short time in strongly radioactive DNA precursor .Then they developed the autoradiogram of the labelled the DNA.
Later experiment with different techniques has shown in E.coli DNA replicates bi-directionally. Experiments in Drosophila and Amphibians also shown that eukaryotic DNA replicates bi-directionally. Unidirectional DNA replication is seen in ColE1 plasmid (E.coli).
Replicon:
The DNA under the control of one origin of replication is called as replicon, the E.coli chromosome is a single replicon, it has only one origin of replication.
Replication fork:
A branch point in a replication eye at which DNA synthesis occurs is called a replication fork. Primase is sensitive to the RNA polymerase inhibitor rifampicin, the observation that rifampicin inhibits only leading strand synthesis therefore indicates that primase initiates the okazaki fragments primers. RNAP or primase both alone can initiates leading strand synthesis but greatly stimulated when both are present. It is therefore thought that these enzymes act synergistically in vivo to prime leading strand synthesis.
Enzymes of DNA replication:
DNA pol I:
In 1957 DNA polymerase I was first identified by Arthur Kornberg. DNA polymerase I is a monomeric enzyme of 928 residues. Pol I couples deoxyribonucleotide triphosphates on DNA templates in reaction that occurs through nucleophilic attack of the growing DNA chain 3-OH group on the a- phosphoryl group of an incoming nucleoside triphosphate. The reaction is driven by the resulting elimination of pyrophosphaye (PPi) and its subsequent hydrolysis by pyrophosphatase.
Error rate is around one wrong base per 10 million, pol I is said to be processive in that it catalyses a series of successive polymerization steps typically 20 or more without releasing the template (opposite of processive or distributive).
Pol I recognizes the incoming dNTPs according to the shape of the base pair it forms with the template DNA. 2, 4 diflouro Toluene base, which is isosteric with thymine but doesn’t accept hydrogen bonds is synthetically inserted in to DNA template, pol I incorporate adenine opposite the 2,4 diflouro toluene.
Pol I has a unique as can edit its mistakes, it can act as both a 3->5 exonuclease and 5->3 exonuclease . Pyrophoshohydrolysis is different from exonuclease, in exonuclease reaction H2O is acceptor of nucleotide rather than ppi.
Pol I acts as the major repair enzyme correcting the mistakes with 3% of correctly incorporated nucleotides are also excised.
Pol I has 5->3 exonuclease activity, binds to single strand DNA nick, cleaves DNA in a base paired region beyond the nick, such the DNA is excised as either mono or oligonucleotide of up to 10 bases residues. The 5->3 exonuclease removes only unpaired mononucleotides with 3-OH groups.
Pol I has two exonuclease functions with each activity occupying separate active sites. The 5->3 Exonuclease activity of pol I is independent of both its 3->5 exonuclease and polymerase activities.
Protease subtilin or trypsin cleaves pol I into 2 fragments, larger c-terminal or klenow fragment (residues 324-928) which contain both 3->5 exonuclease and polymerase activities and a smaller or N terminal fragment (1-323) has 5->3 exonuclease activity. DNA pol I has 3 active sites on single polypeptides (928). It consists of two domains, smaller domain 3->5 exonuclease activity, larger domain polymerase activity. The polymerase domains contain finger, palm and thumb doamins. Finger domain binds to incoming nucleotides and template base, thumb domain that guides the newly formed ds DNA as it leaves active sites. Palm domain contains the active site at bottom of the cleft between finger and thumb domain.
DNA polymerase distinguishes Watson crick base pair via sequence independent interaction that induces domain movement. The 3’ bases of template DNA at active site pocket of DNA pol I assume A- form of DNA. Conformational changes also distinguish correct base pairing. Only when correct base pairing occurs the open confirmation becomes tightly closed confirmation.
The DNA polymerase catalytic mechanism involves two metal ions, pol active sites all contain metal ions, usually Mg+2, that are ligand by two invariant asp side chains in the palm domain.
Formation of Watson and crick base pair facilitates the binding of primer strand to the polymerase site preparatory for the next round of chain extension. Whereas mismatched base pairs greatly show the polymerase reaction while promoting the binding of the primer strand to the exonuclease activity site.
Pol I functions physiologically to repair DNA, the major function is to catalyses the nick translation process.
DNA Pol III:
The discovery of normally growing E.coli mutants that have very little pol I activity stimulated the search for an additional DNA polymerizing activity. DNA pol III act as the major DNA replicase in E.coli. .
DNA Pol II:
It has been implicated as participant in repairing DNA damage via the SOS response along with two additional pol IV and Pol V. 5->3 exonuclease activity is found only in pol I, pol II.
Temperature sensitive pol C mutants above the restrictive temp demonstrate pol III is E.coli replicase. Pol III core has the subunit composition a£ Ø, whereas a, the pol C gene product contain polymerase activity.
A pol C- 5®3’ polymerase
e pol C – Exonuclease activity 3-5
q pol C- stimulates £
i Pol C- dimerizes core and bind to gamma complex
g- binds o ATP
d- binds to beta
ß- Processivity factor- sliding clamp
Pol III has 5->3 exonuclease activity acts only on single stranded DNA, but cannot catalyse at nick translation. Poll III has thumb, finger and palm domain, in addition poll III has an N-terminal PHP (Polymerase and histidinol phosphatase). Poll III core function in vivo as a part of a complicated and labile multiple subunits enzyme, the pol III holoenzyme (subunits). The core enzyme (a,e,?) has a processivity of 10-15% residues , however pol III core is transformed as a highly processive enzyme by association with ß subunit.
The ß subunit confers essentially unlimited processivity (> 5000residues) on the core enzyme. The gamma complex which acts as the clamp loader is subsequently removed. Beta subunit forms a ring like sliding clamp. The interior surface of the protein is positively charged and outer surface is negatively charged.
Helicase and SSBPs:
Three proteins DnaB, rep helicase and SSB proteins unwind the DNA before an under winding replication fork in a process that driven by ATP hydrolysis.
Helicases are involved in replication, recombination, repair and gene expression and other mechanisms like RNA splicing, RNA editing. All life forms have helicases and E.coli has 12 forms of helicases which function by translocating on single stranded of DNA to separate them. Helicase requires ATP hydrolysis. Helicases can translocate in in 5->3 and 3-> 5 directions. DNA B protein helicase is a hexametric protein, that acts by translocating on lagging strand template by hydrolysis of ATP.
Rep helicase drive separation of the strands of DNA via an active rolling mechanism. Rep A protein and pri A protein participate in E.coli DNA replication and both proteins translocate along with DNA in the 3->5 direction (opposite strand from DNA B) while hydrolysing ATP. Single stranded binding protein prevents unwound single stranded DNA from reannealing . It also prevents single stranded DNA from formation of intermolecular secondary structure and protects it from nuclease activity. E.coli SSB is a homodimer of 177 residues subunits. Gp32 and gp2 are SSB of T4 phage and M13 respectively and act cooperatively (binding of one protien facilitate to another another protein).
DNA ligase:
Pol I replaces the RNA primers with DNA through nick translation. Resulting single stranded nicks are sealed by DNA ligase. The free energy required for the reaction is obtained in a species dependent manner through the coupled hydrolysis of either NAD+ to NMN+ + AMP or ATP to ppi+ AMP. E.coli enzyme ligase utilizes NAD+ and Bacteriophage T4 and all eukaryotes ligases use ATP.
Primase:
Primase is a specialized RNA polymerase that has high processivity and less fidelity compound to RNA polymerase. Without primer the DNA replication is error prone and time consuming. Primases from bacteria and phage’s tracks the replication fork in association with its DNA helicases. Helicase moves along on lagging strand in 5’?3’ direction. DnaG protein acts as the primase. Since replication fork moves 1000NTs /sec Primase must synthesize one primer/sec for lagging strand synthesis.
Dna G is a monomer with 581 residues and contains 3 domains.
Mechanism of DNA replication:
Most of the details the DNA replication is obtained from the DNA replication of bacteriophage M13 and ØX174. It occurs in 3 stages - Initiation, Extension and Termination.
Replication Initiation:
E.coli genome is a single covalently closed circular ds DNA (CCC DNA) with a single origin of replication (Ori C). Ori C is a 245bp sequence with 3-13mers AT rich region,5- 9mers region , this also called as Dna A boxes.
DNA A protein recognise and binds to the DNA A boxes with central protein of approximate 30 DNA A copies wrapped by the negatively supercoiled DNA (electron microscopy, DNAse I protection) .
Proteins HU/IHC (A protein that is found always bound to E.coli DNA) facilitates the bending of DNA during this Dna A - Ori C complex formation. Due to the Dna A. DNA interactions the AT rich 13mers, get partially unwinded in presence of ATP. The in vitro experiment showed that this partial unwinding of DNA takes place only above 22°C and in presence of ATP, DNA A protein hydrolyses ATP in a DNA dependent manner. The P1 nuclease assay revealed approximately an 45 bp region of DNA which gets unwinded form an open complex (P1 nuclease cleaves the ss DNA).
Once the single strand regions are formed, the tetramer ssb (single strand binding) protein to ss DNA in a co-operative manner and prevents the rewinding of DNA. Dna B6-C6 (hexameric) protein complex along with gyrase is then joins the open complex. The function of Dna C is only to recruit DNA helicase. The complex of different proteins like DNA helicase, Dna B, Primase and Dna G, together ia refeered as Primosome. It operates repeatedly in priming okazaki fragments to build the lagging strand and Dna B protein serves as the helicase to unwind the DNA (leading and lagging strand)
The DNA Primase is recruited along with DNA pol at the origin converting the pre initiation complex to the priming complex. Similar to RNA pol, Dna G or Dna Primase required SS DNA template, the four types of NTPs, Mg+2 ions using which it synthesizes RNA in 5->3 direction de novo.
11-13NTs RNA primers are synthesized by priming complex due to the simultaneous action of DNA helicase and DNA Primase. The Dna G –RNA polymerase complex is seen in E.coli cells only at the origin replication indicating that the RNA polymerase activates DNA Primase for the primer synthesis. DNA pol III is then recruited at the origin forming a Replication Initiation Complex (RIC). The DNA pol III starts extending the RNA primers which are present on both the parental strands.
Elongation:
The region of DNA at which there is simultaneous unwinding of parental DNA strands and synthesis of new strands is called a replication fork. On each side of the origin, the replication fork moves synthesizing the daughter strand. At each replication fork, the parental DNA strand that runs in 5?3 direction is called Lagging strand template and the other strand which runs in 3?5 direction is called as Leading strand template.
Based on the replication fork movement one of the daughter strand has to be synthesized in 5?3 directions and is called as leading strand, whereas the other one that need 3’?5’ synthesis is called as lagging strand.
During the elongation process, the Primosome complex synthesis multiple RNA primers on the lagging strands at a distance of 1000 NTs, whereas a single RNA primers is synthesized on the leading strand template. A single DNA pol III enzyme that has only 5à3 polymerase activity has to synthesize both leading and lagging strands. It was proved experimentally that the stoichiometry between the number of replication fork and number of DNA pol III enzymes always is 1:1.
For the enzyme like DNA pol III it is highly unlikely to synthesize both leading and lagging strands moving in opposite direction. Two different models were proposed to explain the simultaneously synthesis of leading and lagging strands by a single DNA pol III complex.
Inverted Loop model:
The DNA pol III forms an inverted loop in the lagging strand template by which the orientation of RNA primer will be reversed and its 3’-OH group will now face the replication fork .
The DNA pol III moves in single direction, synthesizing the Okazaki fragments along with the leading strand. Once an Okazaki fragment is synthesized, the loop that is formed is released and the enzyme forms another inverted loop at the next RNA primer and this process continuous till the end of the DNA replication.
Dragging model:
The DNA pol III drags the lagging strand template towards inside through the active site and while dragging it synthesizes the Okazaki fragments and this process is repeated throughout the replication.
The leading and lagging strands present on one side of the origin will become lagging and leading strands respectively on the other side of the origin. During the replication fork progression, the DNA gets positively supercoiled due to unwinding process at the downstream portion. Once the DNA gets saturated with supercoils, further unwinding is not allowed leading to inhibition of replication. DNA Gyrase acts towards the upstream of the replication fork and introduces negative supercoiling to overcome this problem, DNA Topoisomerase I causes the relaxation of negative supercoils at the downstream region.
Termination:
Ter B an F sites acts as backup sites for T and C. Similarly as the replication fork moves in anticlockwise direction, patterns passes through the ter F,B and C will be stopped at ter A,D and ter E acts as backup site for ter A. Special proteins called as Tus protein (Termination Utilisation substance) are found at all the ter sites which impede the movement of replication fork by interacting with Dna B of replication fork thereby inhibiting the replication process.
E.coli cells are viable even when the ter sites are removed from the genome as replication terminates by collision of moving replication fork. The time taken for C and D were always constant (60mins), E.coli cells shows variability is the generation time from 20 mins to more than 10 hours.
Due to presence of multiple forked chromosome, aneuploidies in terms of the gene copy number are observed in E.coli i.e. the genes present near to the origin are found in multiple copies, whereas the gene present away from the origin are found in single copy.
Regulation of DNA replication is be maintained by concentration of DnaA proteins and methylation of GATC sites.
Eukaryotic DNA replication:
Replication in eukaryotes takes place during S- phase of cell cycle. A typical mammalian cell takes 24 hours to complete the cell cycle in which the S- phase takes approximately 6-8 hrs. Time taken for cell cycle in eukaryotes varies from 48 hrs more than 100days and most of this variation is due to time taken by G1 phase.
The details of Eukaryotic replication are mostly obtained for the studies on budding yeast, Fission yeast, SV40 virus, and cells of metazoans like Drosophila and Xenopus.
The major difference between prokaryotic and eukaryotic DNA replication is separation of origin selection and replication initiation events through the formation of pre-replication complex which prevents the over replication of genome. Once the eukaryotic chromatin is opened specific initiator proteins bind to the origins forming Origin replication complexes (ORC).A group of 20-80 origins were to be found to be involved in initiation of replication at first, which after some time progress into the other origin of replication. Some of the origins are not to all utilised during replication. The reinitiating of replication at some origins is completed before the other origins start strictly in eukaryotes due to a Silencing Factor.ORC is ATP regulated and consists of 6 polypeptides (ORC1-6). ORC recruits Cdc 6 (cell division cycle 6) and Mcm (Mini chromosome maintenance) and other accessory proteins.
Replication in eukaryotes is Bi-directional and starts at internal positions at individual origins rather than at at terminal points of linear DNA. In mouse genome 25,000 origins are present and in Humans 10,000-1,00,000 origins have been detected.
The mechanism of DNA replication in eukaryotes involves 12 major steps.
Comparison of proteins involved in replication of E,coli and Humans
Messelson and Stahl Experiment:
The experiment carried out by Meselson and Stahl involved growing a culture of Escherichia coli in a medium containing 15NH4Cl (ammonium chloride labelled with the heavy isotope of nitrogen). The labelled bacterial culture was transferred to a14N containing media and density of their DNA was monitored as a function of bacteria growth by" equilibrium density gradient ultracentrifugation". samples were taken after 20 minutes (one cell division) and 40 minutes (two cell divisions). DNA was extracted from each sample and the molecules were analyzed by density gradient centrifugation. After 20 minutes the entire DNA contained similar amounts of 14N and 15N, but after 40 minutes two bands were seen, one corresponding to hybrid 14N-15N-DNA, and the other corresponding to DNA molecules made entirely of 14N. The predicted outcome of the experiment is shown for each of the three possible modes of DNA replication.
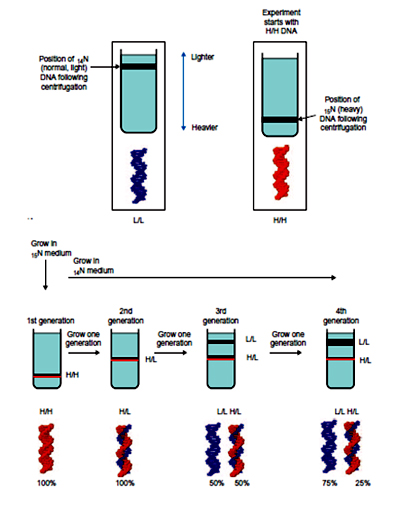
Semi discontinuous mode of DNA replication:
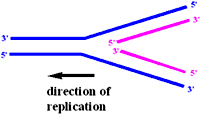
Reiji Okazaki concluded that DNA polymerase could theoretically make one strand continuously and other discontinuously. This discontinuity in DNA synthesis on one of the strand is due to its opposite orientation to the direction in which the replication fork is moving. So this strand is synthesized as fragments one behind the other. Okazaki and his colleagues performed experiments to prove the existence of such DNA fragments during replication. They labelled T4 phage DNA in short pulses and observed DNA pieces at very initial time points of DNA, when these investigators used T4 ligase mutants the pieces remained larger.
Priming of DNA synthesis:
Initiation of DNA synthesis requires a RNA primer, which is further extended by DNA polymerase at its 3' end adding nucleotides. Experimental evidence supporting RNA priming was given when replication of M13 phage in E.coli extracts was inhibited on addition of "rifampicin", an antibiotic that inhibits E.coli "RNAP".
Tuneki Okazaki (wife of Reiji Okazaki) and his co-workers purified 10-12 nucleotides RNA primers. They used mutant bacteria which lack RNAse H or nuclease activity of DNA pol I or both. To label intact primer they used the capping enzyme, gunaylyl transferase and a-32P-GTP to label 5' end of RNAs. After labelling they removed DNA portion with DNAse and subjected to gel electrophoresis which yielded primers of 11-12 nucleotides long in all lanes.
Bi-directional DNA replication:
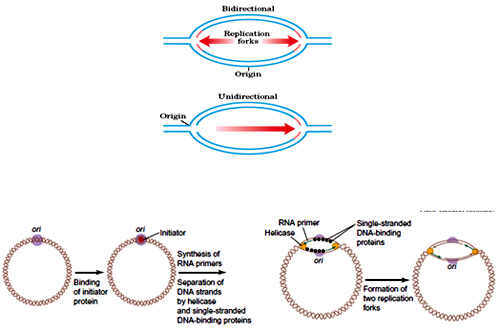
Elizabeth and wake performed experiment on B.subtilis, as they have grown these cells initially in a medium with weakly radioactive DNA precursor followed by short time in strongly radioactive DNA precursor .Then they developed the autoradiogram of the labelled the DNA.
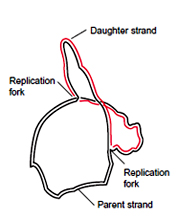
Replicon:
The DNA under the control of one origin of replication is called as replicon, the E.coli chromosome is a single replicon, it has only one origin of replication.
Replication fork:
A branch point in a replication eye at which DNA synthesis occurs is called a replication fork. Primase is sensitive to the RNA polymerase inhibitor rifampicin, the observation that rifampicin inhibits only leading strand synthesis therefore indicates that primase initiates the okazaki fragments primers. RNAP or primase both alone can initiates leading strand synthesis but greatly stimulated when both are present. It is therefore thought that these enzymes act synergistically in vivo to prime leading strand synthesis.
Enzymes of DNA replication:
DNA pol I:
In 1957 DNA polymerase I was first identified by Arthur Kornberg. DNA polymerase I is a monomeric enzyme of 928 residues. Pol I couples deoxyribonucleotide triphosphates on DNA templates in reaction that occurs through nucleophilic attack of the growing DNA chain 3-OH group on the a- phosphoryl group of an incoming nucleoside triphosphate. The reaction is driven by the resulting elimination of pyrophosphaye (PPi) and its subsequent hydrolysis by pyrophosphatase.
Error rate is around one wrong base per 10 million, pol I is said to be processive in that it catalyses a series of successive polymerization steps typically 20 or more without releasing the template (opposite of processive or distributive).
Pol I recognizes the incoming dNTPs according to the shape of the base pair it forms with the template DNA. 2, 4 diflouro Toluene base, which is isosteric with thymine but doesn’t accept hydrogen bonds is synthetically inserted in to DNA template, pol I incorporate adenine opposite the 2,4 diflouro toluene.
Pol I has a unique as can edit its mistakes, it can act as both a 3->5 exonuclease and 5->3 exonuclease . Pyrophoshohydrolysis is different from exonuclease, in exonuclease reaction H2O is acceptor of nucleotide rather than ppi.
Pol I acts as the major repair enzyme correcting the mistakes with 3% of correctly incorporated nucleotides are also excised.
Pol I has 5->3 exonuclease activity, binds to single strand DNA nick, cleaves DNA in a base paired region beyond the nick, such the DNA is excised as either mono or oligonucleotide of up to 10 bases residues. The 5->3 exonuclease removes only unpaired mononucleotides with 3-OH groups.
Pol I has two exonuclease functions with each activity occupying separate active sites. The 5->3 Exonuclease activity of pol I is independent of both its 3->5 exonuclease and polymerase activities.
Protease subtilin or trypsin cleaves pol I into 2 fragments, larger c-terminal or klenow fragment (residues 324-928) which contain both 3->5 exonuclease and polymerase activities and a smaller or N terminal fragment (1-323) has 5->3 exonuclease activity. DNA pol I has 3 active sites on single polypeptides (928). It consists of two domains, smaller domain 3->5 exonuclease activity, larger domain polymerase activity. The polymerase domains contain finger, palm and thumb doamins. Finger domain binds to incoming nucleotides and template base, thumb domain that guides the newly formed ds DNA as it leaves active sites. Palm domain contains the active site at bottom of the cleft between finger and thumb domain.
DNA polymerase distinguishes Watson crick base pair via sequence independent interaction that induces domain movement. The 3’ bases of template DNA at active site pocket of DNA pol I assume A- form of DNA. Conformational changes also distinguish correct base pairing. Only when correct base pairing occurs the open confirmation becomes tightly closed confirmation.
The DNA polymerase catalytic mechanism involves two metal ions, pol active sites all contain metal ions, usually Mg+2, that are ligand by two invariant asp side chains in the palm domain.
Formation of Watson and crick base pair facilitates the binding of primer strand to the polymerase site preparatory for the next round of chain extension. Whereas mismatched base pairs greatly show the polymerase reaction while promoting the binding of the primer strand to the exonuclease activity site.
Pol I functions physiologically to repair DNA, the major function is to catalyses the nick translation process.
DNA Pol III:
The discovery of normally growing E.coli mutants that have very little pol I activity stimulated the search for an additional DNA polymerizing activity. DNA pol III act as the major DNA replicase in E.coli. .
DNA Pol II:
It has been implicated as participant in repairing DNA damage via the SOS response along with two additional pol IV and Pol V. 5->3 exonuclease activity is found only in pol I, pol II.
Temperature sensitive pol C mutants above the restrictive temp demonstrate pol III is E.coli replicase. Pol III core has the subunit composition a£ Ø, whereas a, the pol C gene product contain polymerase activity.
A pol C- 5®3’ polymerase
e pol C – Exonuclease activity 3-5
q pol C- stimulates £
i Pol C- dimerizes core and bind to gamma complex
g- binds o ATP
d- binds to beta
ß- Processivity factor- sliding clamp
Pol III has 5->3 exonuclease activity acts only on single stranded DNA, but cannot catalyse at nick translation. Poll III has thumb, finger and palm domain, in addition poll III has an N-terminal PHP (Polymerase and histidinol phosphatase). Poll III core function in vivo as a part of a complicated and labile multiple subunits enzyme, the pol III holoenzyme (subunits). The core enzyme (a,e,?) has a processivity of 10-15% residues , however pol III core is transformed as a highly processive enzyme by association with ß subunit.
The ß subunit confers essentially unlimited processivity (> 5000residues) on the core enzyme. The gamma complex which acts as the clamp loader is subsequently removed. Beta subunit forms a ring like sliding clamp. The interior surface of the protein is positively charged and outer surface is negatively charged.
Helicase and SSBPs:
Three proteins DnaB, rep helicase and SSB proteins unwind the DNA before an under winding replication fork in a process that driven by ATP hydrolysis.
Helicases are involved in replication, recombination, repair and gene expression and other mechanisms like RNA splicing, RNA editing. All life forms have helicases and E.coli has 12 forms of helicases which function by translocating on single stranded of DNA to separate them. Helicase requires ATP hydrolysis. Helicases can translocate in in 5->3 and 3-> 5 directions. DNA B protein helicase is a hexametric protein, that acts by translocating on lagging strand template by hydrolysis of ATP.
Rep helicase drive separation of the strands of DNA via an active rolling mechanism. Rep A protein and pri A protein participate in E.coli DNA replication and both proteins translocate along with DNA in the 3->5 direction (opposite strand from DNA B) while hydrolysing ATP. Single stranded binding protein prevents unwound single stranded DNA from reannealing . It also prevents single stranded DNA from formation of intermolecular secondary structure and protects it from nuclease activity. E.coli SSB is a homodimer of 177 residues subunits. Gp32 and gp2 are SSB of T4 phage and M13 respectively and act cooperatively (binding of one protien facilitate to another another protein).
DNA ligase:
Pol I replaces the RNA primers with DNA through nick translation. Resulting single stranded nicks are sealed by DNA ligase. The free energy required for the reaction is obtained in a species dependent manner through the coupled hydrolysis of either NAD+ to NMN+ + AMP or ATP to ppi+ AMP. E.coli enzyme ligase utilizes NAD+ and Bacteriophage T4 and all eukaryotes ligases use ATP.
Primase:
Primase is a specialized RNA polymerase that has high processivity and less fidelity compound to RNA polymerase. Without primer the DNA replication is error prone and time consuming. Primases from bacteria and phage’s tracks the replication fork in association with its DNA helicases. Helicase moves along on lagging strand in 5’?3’ direction. DnaG protein acts as the primase. Since replication fork moves 1000NTs /sec Primase must synthesize one primer/sec for lagging strand synthesis.
Dna G is a monomer with 581 residues and contains 3 domains.
- Zn+2 binding N-terminal domain
- RNAP domain
- C-terminal domain-helicase binding domain.
Mechanism of DNA replication:
Most of the details the DNA replication is obtained from the DNA replication of bacteriophage M13 and ØX174. It occurs in 3 stages - Initiation, Extension and Termination.
Replication Initiation:
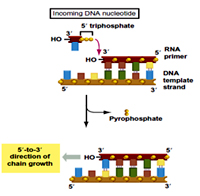
DNA A protein recognise and binds to the DNA A boxes with central protein of approximate 30 DNA A copies wrapped by the negatively supercoiled DNA (electron microscopy, DNAse I protection) .
Proteins HU/IHC (A protein that is found always bound to E.coli DNA) facilitates the bending of DNA during this Dna A - Ori C complex formation. Due to the Dna A. DNA interactions the AT rich 13mers, get partially unwinded in presence of ATP. The in vitro experiment showed that this partial unwinding of DNA takes place only above 22°C and in presence of ATP, DNA A protein hydrolyses ATP in a DNA dependent manner. The P1 nuclease assay revealed approximately an 45 bp region of DNA which gets unwinded form an open complex (P1 nuclease cleaves the ss DNA).
Once the single strand regions are formed, the tetramer ssb (single strand binding) protein to ss DNA in a co-operative manner and prevents the rewinding of DNA. Dna B6-C6 (hexameric) protein complex along with gyrase is then joins the open complex. The function of Dna C is only to recruit DNA helicase. The complex of different proteins like DNA helicase, Dna B, Primase and Dna G, together ia refeered as Primosome. It operates repeatedly in priming okazaki fragments to build the lagging strand and Dna B protein serves as the helicase to unwind the DNA (leading and lagging strand)
The DNA Primase is recruited along with DNA pol at the origin converting the pre initiation complex to the priming complex. Similar to RNA pol, Dna G or Dna Primase required SS DNA template, the four types of NTPs, Mg+2 ions using which it synthesizes RNA in 5->3 direction de novo.
11-13NTs RNA primers are synthesized by priming complex due to the simultaneous action of DNA helicase and DNA Primase. The Dna G –RNA polymerase complex is seen in E.coli cells only at the origin replication indicating that the RNA polymerase activates DNA Primase for the primer synthesis. DNA pol III is then recruited at the origin forming a Replication Initiation Complex (RIC). The DNA pol III starts extending the RNA primers which are present on both the parental strands.
Elongation:
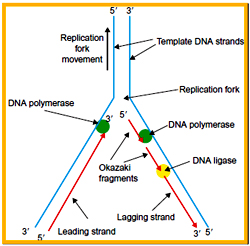
Based on the replication fork movement one of the daughter strand has to be synthesized in 5?3 directions and is called as leading strand, whereas the other one that need 3’?5’ synthesis is called as lagging strand.
During the elongation process, the Primosome complex synthesis multiple RNA primers on the lagging strands at a distance of 1000 NTs, whereas a single RNA primers is synthesized on the leading strand template. A single DNA pol III enzyme that has only 5à3 polymerase activity has to synthesize both leading and lagging strands. It was proved experimentally that the stoichiometry between the number of replication fork and number of DNA pol III enzymes always is 1:1.
For the enzyme like DNA pol III it is highly unlikely to synthesize both leading and lagging strands moving in opposite direction. Two different models were proposed to explain the simultaneously synthesis of leading and lagging strands by a single DNA pol III complex.
Inverted Loop model:
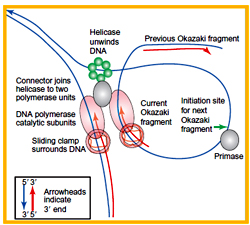
The DNA pol III moves in single direction, synthesizing the Okazaki fragments along with the leading strand. Once an Okazaki fragment is synthesized, the loop that is formed is released and the enzyme forms another inverted loop at the next RNA primer and this process continuous till the end of the DNA replication.
Dragging model:
The DNA pol III drags the lagging strand template towards inside through the active site and while dragging it synthesizes the Okazaki fragments and this process is repeated throughout the replication.
The leading and lagging strands present on one side of the origin will become lagging and leading strands respectively on the other side of the origin. During the replication fork progression, the DNA gets positively supercoiled due to unwinding process at the downstream portion. Once the DNA gets saturated with supercoils, further unwinding is not allowed leading to inhibition of replication. DNA Gyrase acts towards the upstream of the replication fork and introduces negative supercoiling to overcome this problem, DNA Topoisomerase I causes the relaxation of negative supercoils at the downstream region.
Termination:
Ter B an F sites acts as backup sites for T and C. Similarly as the replication fork moves in anticlockwise direction, patterns passes through the ter F,B and C will be stopped at ter A,D and ter E acts as backup site for ter A. Special proteins called as Tus protein (Termination Utilisation substance) are found at all the ter sites which impede the movement of replication fork by interacting with Dna B of replication fork thereby inhibiting the replication process.
E.coli cells are viable even when the ter sites are removed from the genome as replication terminates by collision of moving replication fork. The time taken for C and D were always constant (60mins), E.coli cells shows variability is the generation time from 20 mins to more than 10 hours.
Due to presence of multiple forked chromosome, aneuploidies in terms of the gene copy number are observed in E.coli i.e. the genes present near to the origin are found in multiple copies, whereas the gene present away from the origin are found in single copy.
Regulation of DNA replication is be maintained by concentration of DnaA proteins and methylation of GATC sites.
Eukaryotic DNA replication:
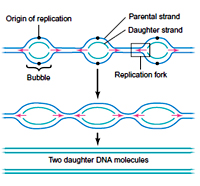
The details of Eukaryotic replication are mostly obtained for the studies on budding yeast, Fission yeast, SV40 virus, and cells of metazoans like Drosophila and Xenopus.
The major difference between prokaryotic and eukaryotic DNA replication is separation of origin selection and replication initiation events through the formation of pre-replication complex which prevents the over replication of genome. Once the eukaryotic chromatin is opened specific initiator proteins bind to the origins forming Origin replication complexes (ORC).A group of 20-80 origins were to be found to be involved in initiation of replication at first, which after some time progress into the other origin of replication. Some of the origins are not to all utilised during replication. The reinitiating of replication at some origins is completed before the other origins start strictly in eukaryotes due to a Silencing Factor.ORC is ATP regulated and consists of 6 polypeptides (ORC1-6). ORC recruits Cdc 6 (cell division cycle 6) and Mcm (Mini chromosome maintenance) and other accessory proteins.
Replication in eukaryotes is Bi-directional and starts at internal positions at individual origins rather than at at terminal points of linear DNA. In mouse genome 25,000 origins are present and in Humans 10,000-1,00,000 origins have been detected.
The mechanism of DNA replication in eukaryotes involves 12 major steps.
- Histones are removed allowing access to replication machinery.
- Formation of pre-replication complex.
- Replication ’licensing’, once bound ORC recruits at least two different proteins, Cdc6 and Cdt1.ORC and then two proteins recruit Mcm2-7 helicase complex to formation pre- replication complex. Cell cycle regulation of pre replication complex and activation ensures that DNA replicates only once per cell cycle.
- Duplex unwinding at replication forks and relaxation of positive supercoils is mediated after the release of Cdc6 and Cdt1 from the complex nad replication factors like RP-A are recruited. The helicase activity of Mcm 2-7.unwinds the DNA duplex. Topoisomerase I/II resolves the supercoils ahead of the replication fork.
- RNA priming of leading and lagging strands is done by primase which is in association with DNA polymerase which is subsequently extended by the DNA polymerase
- The resulting primer-template junction is recognised by the sliding clamp loader (RFC) which assembles a sliding clamp, PCNA at the sites. Either DNA polymerase d or DNA polymerase e recognises the primer and begins leading or lagging strand synthesis This strategy of employing a different polymerase after initiation is called Polymeraese Switching.
- Elongation of leading and lagging strands is done by the respective polymerases .in 5’®3’ direction.
- Continuous synthesis occurs on leading strand and polymerase switching occurs on the lagging strand.
- Removal of RNA primers is done by FEN-1 which degrades them by endonuclease activity.
- Filling in of gaps generated by the removal of the primers is done by either DNA polymerase d or DNA polymerase e.
- Joining of okazaki fragments is done by DNA ligase I with the aid of PCNA.
- Histone deposition for nucleosome formation is mediated by the action of CAF-1 (Chromatin Assembly Factor-1) and PCNA
- Pol a: acts as a primase (synthesizing a RNA primer), and then as a DNA Pol elongating that primer with DNA nucleotides. After around 20 nucleotides elongation is taken over by Pol d (on the lagging strand) and e (on the leading strand).
- Pol ß: is implicated in repairing DNA.
- Pol g: replicates mitochondrial DNA
- Pol d: is the main polymerase on the lagging strand in eukaryotes, it is highly processive and has 3'®5' exonuclease activity.
- Pol e: is the primary leading strand DNA polymerase in eukaryotes, and is also highly processive and has 3'->5' exonuclease activity.
- h, i, k, and Rev1 are Y-family DNA polymerases and Pol z is a B-family DNA polymerase. These polymerases are involved in the bypass of DNA damage.
Comparison of proteins involved in replication of E,coli and Humans
Proteins | E.coli | Humans |
Helicase | DNA B | Mcm 2-7 (T-antigen) |
Loading Helicase/Primase | DNA C | Mcm 2-7 |
Single strand maintenance | ssb | RPA |
Priming | DNA G | Polymerse / primase |
Sliding clamp | ß | PCNA |
Clamp loader (ATPase) | complex | RFC |
Strand elongation | Polymerase III | Polymerase d/e |
RNA primer removal | Polymerase I | FEN-I-Rnase H |
Ligation of okazaki fragments | Ligase | Ligase I |
Published date : 25 Jun 2014 01:06PM