Chromatin Organisation
Sakshi Education
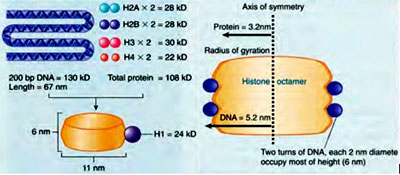
What is Chromatin ?
Chromatin refers to nucleoprotein complexes of DNA and its associated proteins consisting of histones and non-histones. It is often used synonymously with chromosome. Most of the living organisms contain DNA as genetic material. This DNA in the cells is bound by an array of proteins and in fact it is rarely available as free DNA. A set of all DNA sequences present in a cell is called as a Genome. In case of prokaryotes, the genome is present as a single covalently closed circular DNA molecule, whereas in case of eukaryotes genome is present in the form of multiple, discrete independent segregation units called as Chromosomes. The discovery that chromosomes are readily stained by certain dyes greatly facilitated light microscopic studies and led W.Waldeyer in 1888 to coin the word ‘chromosome’, from ‘chromo’(colour) and ‘soma’(body). Although the two terms -chromatin and chromosomes are often used interchangeably, chromosomes are technically representing a highly condensed form of chromatin which appears only during cell division or more specifically during mitotic phase of the cell cycle. During interphase the chromatin is relatively less condensed and exhibits different degrees of condensation.
The need for Chromatin Organization:
The human genome size is 3.1 X 109 bp. DNA and under physiological conditions assumes B-form which occupies 34 A? for 10 bp. If we calculate the total length of the DNA it amounts to 1 metre approximately for haploid DNA content. But the size of the nucleus in which it is accommodated is in the range of 10 µm. To put this into perspective, imagine a magnifying a cell 10 million times to the size of a football stadium with the nucleus the size of the pitch. On this scale, DNA would be like a rope 2cm in diameter and 20,000 km long- a length that would stretch halfway around the world. Therefore in order to accommodate such a large sized DNA into much smaller nucleus or a cell or a viral coat, it needs to be condensed to a large extent. Since DNA acts the genetic material that codes for different proteins and RNA transcripts, it should be selectively available for transcription inspite of being condensed. Therefore the process of condensation should be carried out in a defined manner such that desirable regions can be accessed by the cell at any given time. In addition condensation of DNA is a cumbersome process as it is polyanionic in nature bearing a continuous stretch of negative charge all over helix. Due to the repulsions between the negatively charged phosphate groups of the DNA, condensation cannot be carried out without the neutralization of negative charges. Charge neutralization is done by binding of positively charged proteins called histones. histones are absent in prokaryotes but histone like proteins are present. Unlike histones these proteins are redundant in nature. Any mutation in the genes encoding histones is found to be lethal. In fact histones are one of the most conserved genes and are present in the form of operons in the genome and histone genes lack introns. Mutations in Hu and Hns type of histone like proteins in prokaryotes are not lethal. A function lost due to mutation in one gene can restored by other genes. In addition, prokaryotes contain poly amines like spermine and spermidine that bind to DNA for charge neutralization.
Histones - form the basic tools for chromatin organization:
In eukaryotes there are five major types of Histones - H1, H2A, H2B, H3, H4.
H1 is the hypervariable histone and is least conserved. H1 is absent in yeast Saccharomyces cerevisiae. H2A and H2B are moderately conserved and H3 and H4 are highly conserved. Two copies of H2A, H2B, H3 and H4 each combine to form a histone octamer. H1 is not a part of histone octamer can be easily dissociated. Histone octamer forms the cylindrical structure around which DNA binds. H1 seals the DNA wound around the histone octamer and is therefore called linker histone. In this manner DNA is condensed by binding around the basic (positively charged) proteins – Histones, which forms the basis for chromatin organization. Packing Ratio – Levels of Chromatin Organisation: Condensation of DNA varies spatially and temporally and exhibits different .degrees of condensation which can be measured by Packing Ratio or Packing Fraction. Packing Fraction is the ratio of the actual length of the DNA (in fully extended form) to the length of the unit which contains it (condensed from). For instance, the size of the smallest human chromosome is 4.6 x 107 and is 10 times larger than the genome of E.coli and equals to 14,000 µm (1.4cm). In its most condensed form it occupies a length of 2 µm. Thus the packing ratio for chromosome is 7000. Similarly, the largest human chromosome accommodates DNA of about 500 million base pairs, occupying 17cm in length in its extended form exhibiting a packing ratio of 10,000 times. Thus, depending on the degree of condensation, there are three different levels of chromatin organization..
As mentioned earlier histone octamer acquires a cylindrical strutcure which is 6 nm in height and 10.5 nm in width. DNA is wrapped around the cylindrical octamer by taking approximately 1.65 turns. The amount of DNA bound in the centre is more than the amount of DNA bound towards the edges. This feature of allowing more DNA to be wound in the centre leads to a phenomenon called negative supercoiling, which is highly essential for molecular mechanisms like transcription and replication. Initially, Micrococccal nuclease digestion experiments revealed approximately 200bp of DNA wound to histone octamer. But over incubation of the nuclease with the chromatin further yielded 165 bp and at last 146bp of DNA. This 146 bp of DNA is called the Core DNA and the rest of the DNA is called the linker DNA. The length of the linker DNA varies form 8-114bp. Histone octamer together with the core DNA is termed as Nucleosome which is the fundamental unit of chromatin organization. This is the primary level of chromatin organization and is also termed as the 10 nm fibre or nucleosomal model. This structure is obtained when chromatin is isolated under low ionic strength conditions and high temperature. This is more commonly known as "Beads on a string” structure and is rarely found in vivo The Packing Fraction for this level is 6.
30 nm fibre
This is the secondary level of chromatin organization and is also termed a Solenoid model. Each solenoid consists of six nucleosomes along with the linker DNA and H1 HIstones. A Nucleosome along with the H1 and and linker DNA is termed as the Chromatosome. Six chromatosomes constitute a solenoid. This type of structure is obtained when chromatin is isolated under high ionic strength conditions and low temperature and is most likely found at interphase nucleus in vivo.The linking number for this level is 40.
Higher order structures:
Further condensation of 30 nm fibre results in higher order structures whic exhibit diefferent states of condensation at mitotic and interphase,. At the end of mitosis, as the cells enters the interphase, the envelope reforms around the chromatin and chromosomes decondense. Some chromatin does not decondense at the end of mitosis but remains highly compacted. This densely packed chromatin displaying a condition comparable to the mitotic chromosomes is called Heterochromatin which exhibits a packing ratio of 10000 both at interphase and during mitosis. It is recognisable in interphase nuclei by light or electron microscopy and is usually located at the nuclear periphery. Heterochromatin is darkly stained and appears different from the other region and hence the name ‘hetero’ meaning ‘different’ which is primarily of two types- Constitutive and Facultative. Constitutive heterochromatin is mainly found at centromeres and telomeres, consisting primarily of highly repetitive DNA sequences. This region of chromatin always exist in heterochromatic stae and are hence considered to be permanently inactive. It also tends to repress the activity of nearby genes in a process called “Position effect or position effect variegation.
Facultative heterochromatin refers to regions of euchromatin that are converted to heterochromatin. This region contains genes, but their activity is repressed by histone methylation or small interfering RNA (siRNA). An example of facultative heterochromatin is the inactive X-chromosome in human female cells.
By contrast, euchromatin decondenses during interphase. The distinction between heterochromatin and euchromatin was originally based on different staining properties in light microscopy, but the most significant difference is that euchromatin is a transcriptionally active region as it is less condensed and contains active genes while heterochromatin does not. In the later case dislodging of histones and unfolding of DNA strands becomes a cumbersome task.
Chromosome condensation at Mitosis amd the role of Condensin and RCA:
Higher levels of chromatin organization as in the case of mitotic chromosomes exhibit the highest degree of condensation witha packing fraction of 10.000 involve the role of non histone proteins like SMC proteins, Condensins and RCA apart from histones. Genetic screens for chromosome segregation mutants revealed a family of ‘structural maintenance of chromosomes’(SMC) proteins. The condensin complex, which includes components from this family, was identified as a scaffold component and was shown to be necessary for chromosome condensation. More recent studies using condensin mutants and knockdowns show that the chromosome condensation programme has two distinct components. One involves compaction of the chromatin at the onset of mitosis to make the characteristic rod-shaped mitotic chromosomes. The other involves establishment of a robust architecture that allows the chromosome to withstand the spindle forces during metaphase and maintains the characteristic chromosome structure after cyclin-dependent kinase (Cdk) activity falls during anaphase. Several studies have now shown that condensin may not be required for the first component of chromosome condensation, but it is essential for the second.
Recently, a new activity has been suggested for the first component of the chromosome condensation programme: regulator of chromosome architecture (RCA).
It is proposed that RCA drives chromatin condensation at mitosis and that it can do so in the absence of condensins. Its activity is sustained by Cdk1 and it is essential in prometaphase and metaphase. Inactivation of
RCA occurs at anaphase onset and is mediated by a complex of protein phosphatase 1 (PP1) and Repo-Man that is activated at anaphase. Phosphorylation of Repo-Man by Cdk1 prevents its binding to the chromosomes during early mitosis, but its de-phosphorylation activates PP1 and brings the complex to the anaphase chromosomes where it dephosphorylates RCA, triggering chromosome decondensation.
Specialised Chromosome Regions
Some regions of the chromosome appear distinct and can associated with performing specialized functions.For instance, centromere and kinetochore are involved in the proper segregation of chromatids during mitosis, and the telomeres aid in safeguarding the terminal regions of the chromosomes during DNA replication. These structures coordinate in transferring the genetic information from one generation to the other ,with high fidelity. Similarly, the nucleolar organising region (NOR) is the site of ribosomal ribonucleic acid (rRNA) synthesis and assembly of ribosomes.
Centromeres
The term centromere is named based on its location, central’ (centro) ‘region’ (mere). Walter Flemming first identified the region in 1880 as the region where the chromosome is constricted (the primary constriction). It is the region of the chromatin where the sister chromatids are held together until segregation occurs and where the kinetochore assembles providing the structure necessary for attachment of spindle microtubules. Although the name indicates that the location as central but it does not hold true in all the cases and also that not all centromeres can be represented as localised regions. For example, in the nematode Caenorhabditis elegans, the centromere is spread all along the surface of the chromosome. Despite such morphological variations, the basic structure and function of the centromere are highly conserved among species. This region is essential for the survival of cells, and alterations in its structure can cause severe problems during cell division and generate cells with abnormal chromosome number leading to aneuploidy.
The molecular nature of the centromere consists of specialized proteins that are localized to this region. Till date, there are 19 such proteins that are named as CENP for ‘CENtromeric Protein’ including the first three proteins discovered in the series, CENP-A, CENP-B and CENP-C. The presence of these proteins at the centromere can be noticed all throughout the cell cycle, and are involved in forming a network known as CCAN (Constitutive Centromere Associated Network) or CENP-A NAC/CAD (CENP-A Nucleosome Associated Complex/CENP-A Distal complex). CENP-A is the fundamental protein behind the formation of network which is a H3 variant that is found only within the centromeric chromatin. This unique organization provides a base for flexible localised chromatin appropriate for for the assembly of centromeric protein complexes,especially aid in the formation of kinetochore during mitosis. In yeast centromeres have a defined DNA sequence, whereas human centromeres are composed of a highly repetitive, divergent set of tandemly repeated sequences (satellite DNA).
The centromeric chromatin plays a very crucial biological role acting as the physical link between the replicated sister chromatids until they are separated at anaphase. Cohesion are the proteins that are mainly responsible for maintaining the link. Cohesion forms a a pentameric complex similar in composition to the condensin complex . Cohesin makes a ring that is believed to trap two DNA molecules and thereby create a physical connection between sister chromatids. At anaphase onset, the cohesin ring is cleaved by a protease called separase, and the two sister chromatids are free to separate. Another important function of centromeric chromatin is to provide rigidity to oppose the pulling forces generated by spindle microtubules attached to the sister kinetochores. Rigidity can be attributed partially to Cohesin and partially by Condensins. Absence of either of the proteins leads to loss of rigidity.
Kinetochores
Formation of Kinetochores occurs when cells enter mitosis as a consequence of assembly of numerous proteins on the surface of the centromeres. Electron microscopic studies of vertebrate kinetochores reveal a tri-laminar structures with two electron-dense regions (the inner and outer plates) separated by a less dense middle layer. Centromeric proteins are localised in the inner plate, whereas the outer plate contains proteins that interact with microtubules and proteins that are involved in the spindle assembly check point.
Kinetochores act as the molecular machines that not only mediate the attachment of microtubules to the sister chromatids but also verify the appropriateness of the attachments. It ensures that sister chromatids are attached to spindle microtubules emanating from opposite poles and also promotes the correction of improper attachments. In case of any corrections are needed, the anaphase is delayed until all kinetochores are correctly attached to spindle microtubules. A remarkable feature of kinetochores is that they can maintain attachment even to microtubules that are growing or disassembling.
Any error in the attachment would lead to improper segregation of sister chromatids and in order to prevent this the cell division must be stopped at that particular point. Kinetochores mediate their very essential ‘DON’T GO’ signal and is also referred to as the spindle assembly checkpoint.
Telomeres
DNA sequences present at the distal ends of chromosomes are defined as Telomeres. As in the case of eukaryotes, chromosomal DNA molecules are linear and because DNA polymerase requires an RNA primer with a 3’-hydroxyl donor group to initiate DNA replication, some nucleotides are invariably lost from the 5’ end of a DNA strand at each cell division.
To prevent this gradual loss of sequences from the ends and eventually leading to loss of important genes, telomeres are employed to prevent the process, which have a special structure and a mechanism to maintain that structure. Telomeres consist of repetitive DNA sequences, for example, in humans, hundreds to thousands of TTAGGG tandem repeats. This DNA is mostly double-stranded but has a single-stranded terminus 130–210 bases long that can fold on itself and invade the duplex telomeric DNA, resulting in the formation of a telomere loop (T loop). Telomeric DNA is also coated by a capping protein called shelterin that prevents the linear DNA molecule from being recognized by the DNA repair enzymes as a broken molecule in need of repair.
Although some repeats are inevitably lost from the 5’ end during each cycle of replication, the RNA-containing enzyme telomerase can replace them. After DNA replication has occurred, the reverse transcriptase activity of telomerase (TERT) synthesizes telomeric DNA repeats de novo using the RNA component of telomerase (TERC) as a template. When they become too short, telomeres signal the arrest of cell proliferation, senescence and apoptosis. Most somatic human tissues do not have sufficient telomerase activity to prevent telomere shortening; therefore, after several divisions, they will become senescent.
Nucleolar organising regions
NORs are clusters of ribosomal DNA (rDNA) repeats that become the sites of rRNA synthesis and ribosome assembly during interphase. In human cells, they are located on the short arms of the five acrocentric chromosomes (chromosomes 13, 14, 15, 21 and 22) in a telomere-to-centromere orientation . Most human NORs consist of approximately 70 copies of rDNA repeats and contain only rDNA. Active NORs remain undercondensed during mitosis, and they appear as secondary constrictions on metaphase chromosomes. Transcription of the genes in the regions ensures rapid resumption of ribosome biogenesis at the beginning of G1 phase.
Chromatin is present as loops and domains.
Individual chromosomes are not distinguishable in interphase nuclei. Interphase chromosomes also maintain their identity and occupy distinct ‘territories’ within the nucleus rather than becoming all ‘jumbled’ together. Although there are no internal membranes within the nucleus, but territories may be maintained by temporary attachment to filaments (nuclear matrix) that disassemble at the next mitosis. Chromatin loops anchored at their bases to the scaffold, are a feature of mitotic chromosomes. It is likely that such loops, anchored to an interphase scaffold or matrix, also persist during interphase. It has been proposed that these loops could serve as regulatory units for groups of genes, with each loop comprising of a domain in which gene expression is regulated independently of other such domains. Similar pattern is seen in the case of prokaryotes where the chromatin is attached to the plasma mebrane forming loops which act as domains.
Chromatin Remodelling:
Transcription is a process of RNA synthesis using single stranded DNA as template. Generation of single stranded DNA from double stranded requires DNA melting which is accomplished by RNA polymerase and its accessory proteins. But access to DNA for these proteins is possible only when histones bound to DNA are removed. At the same time, after accomplishment of transcription, the naked DNA must be packed again into the chromatin form to restore the original state. This dynamic behavior of chromatin to get dispensed with histones for transcription or replication and rearranging itself back into chromatin form with the assistance of various proteins, is termed as “Chromatin Remodelling”. Chromatin remodeling occurs by means of two pathways – A Replication independent pathway (occurs during transcription) and a replication coupled pathway (occurs during DNA replicatiomn). In RI pathway, reassembly of histone octamers and nucleosome formation involves incorporation of histone variants. For instance, H3.3 is a variant of H3 differing in four amino acids is preferentially incorporated into nucleosomes. This preferential bias towards H3.3 is based on its availability in the cell during interphase as in it happens in the case of the protozoan, Tetrahymena or it can be selectively picked up based on its sequence variation as in the case of Drosophila. In contrast Repilcation coupled pathway does not discriminate between H3 and H3.3 and can incorporate both but on most occasions H3.3 is available in low concentrations and therefore is represented less in the final assembly. Replication of centromeric DNA and reassembly utilizes a protein CENP-A instead of H3. Chromatin reassembly in RC pathway is linked to the replication apparatus, and requires the aid of an ancillary protein CAF-1(Chromatin assembly factor-1), which is recruited to the replication fork by another protein PCNA (Proliferating Cell Nuclear Antigen) which acts as the processivity factor for DNA polymerase.
Organization of Histones
Histone octamers have a kernel of H32-H42 tetramer associated with a dimer of H2A-H2B. The H32-H42 tetramer accounts for the diameter of the octamer and forms the core. H2A-H2B is placed on top and bottom of the tetrameric core. All the four core HIstones exhibit a characterstic structure termed as "Histone fold", with 3 alpha helices connected by two loops with the N terminal ends of all the Histones protruding outside available for different types of modifications responsible for chromatin remodeling.
Histone Modifications
The-protruding N-terminal tails, as well as some amino acids present in the carboxy-terminal part of the histones are subject to various post-translational modifications. These modifications, present individually on the nucleosome or in combination with others (constituting the histone code),together with DNA methylation as seen specifically in mammal act as determinants for the type of chromatin formed- either silent oe active.
Histone acetylation:
Modifications of histones responsible for controlling transcription and thereby gene expression was first demonstrated in ciliates by characterization of the first histone modifying enzymes, a histone acetyl transferase (HAT). .Histone acetylation that occurs at specific lysines on the four core histones, neutralizes the positive charge of the target lysine, thus altering histone–DNA interaction. This creates more ‘open’ chromatin architecture and facilitates the binding of proteins containing bromo domains, which can induce chromatin remodelling, or favour the recruitment of transcription factors necessary for the assembly of the transcriptional machinery. Thus acetylation of histones causes transcriptional activation.
Most familiar example that histone acetylation causes transcriptional activation of genes can be witnessed in Drosophila wherein a global upregulation of genes is achieved on single X chromosomes of males so as to meet the expression levels achieved by two X chromosomes in females. Thus transcriptional activation of genes through histone acetylation is used as a mechanism to enforce Dosage Compensation. A global acetylation of lysine16 of histone H4, observed on the single X-chromosome of males is crucial to this global upregulation of transcription. In general transcriptional activation is a transient phenomenon and repression of transcription is usually established back, making Acetylation, a reversible process.Therefore deacetylation becomes compulsory and is executed by different classes of histone deacetylases (HDACs).Alterations in the histone acetylation plays a key role in normal cell growth is dependent on the dynamic interplay of HATs and HDACs. A number of inhibitors of HDACs are currently used as anticancer agents and others are under clinical evaluation.
Histone methylation
Unlike acetylation, histone methylation does not modify the charge of nucleosomes but influences the docking sites for protein partners. Methylation of occurs on lysine (K) and arginine (R) residues but is predominantly on arginine as is evident from the fact that there are eleven arginine methyl transferases that have been characterized in mammals till date and are classified into two major types.
Type I enzymes catalyze asymmetrical dimethyl arginine and type II enzymes generate symmetrical dimethyl arginine. The potential functional activity of arginine methylation depends on the residue being modified and the type of methylation introduced. Symmetrical and asymmetrical methylation of the arginine3 on histone H4 exhibit opposite effects on transcription regulation. Indeed, whereas PRMT1-mediated asymmetrical methylation correlates with transcriptional activation through a specific interplay with histone acetylation,PRMT5-mediated symmetrical methylation is linked to transcriptional repression. Similar to acetylation, arginine methylation also needs to be dynamically regulated. Two types of enzymes are responsible for demethyaltion on arginine- PAD-4 and JMJD6.
Apart from arginine methylation, histone methyl transferases cause different degrees of lysine methylation (mono-,di-,tri-) that strongly potentialize the cellular interpretation of each lysine methylation mark. By and large methylation leads to condensation of chromatin but depending on the degree of methylation, methylation marks, even on the same histone, could activate transcription or in contrast, participate in the formation of condensed structures. For instance trimethylation of the lysine4 on histone H3 (H3K4me3) is essential for transcriptional activation,whereas trimethylation of lysine9 or of lysine 27on H3 (H3K9me3, H3K27me3) favours the chromatin condensation. In the latter case,H3K9me3 and H3K27me3 marks attract the structural chromodomain-containing proteins HP1and polycomb (PC) responsible for the formation of constitutive and facultative heterochromatin, respectively. Until recently, methylation of lysines was considered to be an irreversible mark but with the discovery of specific lysine demethylases like LSD1, has been now demonstrated that lysine methylation can be dynamically regulated.
Histone phosphorylation:
Histone phosphorylation causes condensation of mitotic chromosomes and chromosome segregation. The phosphorylation of histone H3 at serine10 generates a epigenetic mark which appears at two distinct cell-cycle stages- at G2 and immediate-early response of mammalian cells to mitogens. In the case of chromosome condensation in mitosis, together with H3Ser10P,other phosphorylated marks on the serine 28 and on threonine 11 of histone H3 facilitate the recruitment of condensins and subsequent chromosome segregation.
Histone ubiquitination
Ubiquitination of proteins plays a significant role in a plethora of cellular processes including protein degradation, cell cycle regulation, protein trafficking, stress response, signal transduction and transcriptional regulation, Similarly, ubiquitination of histones primarily occurs on two sites - H2B ubiquitination on lysine 123 and H2A ubiquitination, which, occurs on lysine 119. The ubiquitin moeity can be removed by a large number of deubiquitinating enzymes (DUB) that can have a balancing function for transcriptional regulation.
Chromatin refers to nucleoprotein complexes of DNA and its associated proteins consisting of histones and non-histones. It is often used synonymously with chromosome. Most of the living organisms contain DNA as genetic material. This DNA in the cells is bound by an array of proteins and in fact it is rarely available as free DNA. A set of all DNA sequences present in a cell is called as a Genome. In case of prokaryotes, the genome is present as a single covalently closed circular DNA molecule, whereas in case of eukaryotes genome is present in the form of multiple, discrete independent segregation units called as Chromosomes. The discovery that chromosomes are readily stained by certain dyes greatly facilitated light microscopic studies and led W.Waldeyer in 1888 to coin the word ‘chromosome’, from ‘chromo’(colour) and ‘soma’(body). Although the two terms -chromatin and chromosomes are often used interchangeably, chromosomes are technically representing a highly condensed form of chromatin which appears only during cell division or more specifically during mitotic phase of the cell cycle. During interphase the chromatin is relatively less condensed and exhibits different degrees of condensation.
The need for Chromatin Organization:
The human genome size is 3.1 X 109 bp. DNA and under physiological conditions assumes B-form which occupies 34 A? for 10 bp. If we calculate the total length of the DNA it amounts to 1 metre approximately for haploid DNA content. But the size of the nucleus in which it is accommodated is in the range of 10 µm. To put this into perspective, imagine a magnifying a cell 10 million times to the size of a football stadium with the nucleus the size of the pitch. On this scale, DNA would be like a rope 2cm in diameter and 20,000 km long- a length that would stretch halfway around the world. Therefore in order to accommodate such a large sized DNA into much smaller nucleus or a cell or a viral coat, it needs to be condensed to a large extent. Since DNA acts the genetic material that codes for different proteins and RNA transcripts, it should be selectively available for transcription inspite of being condensed. Therefore the process of condensation should be carried out in a defined manner such that desirable regions can be accessed by the cell at any given time. In addition condensation of DNA is a cumbersome process as it is polyanionic in nature bearing a continuous stretch of negative charge all over helix. Due to the repulsions between the negatively charged phosphate groups of the DNA, condensation cannot be carried out without the neutralization of negative charges. Charge neutralization is done by binding of positively charged proteins called histones. histones are absent in prokaryotes but histone like proteins are present. Unlike histones these proteins are redundant in nature. Any mutation in the genes encoding histones is found to be lethal. In fact histones are one of the most conserved genes and are present in the form of operons in the genome and histone genes lack introns. Mutations in Hu and Hns type of histone like proteins in prokaryotes are not lethal. A function lost due to mutation in one gene can restored by other genes. In addition, prokaryotes contain poly amines like spermine and spermidine that bind to DNA for charge neutralization.
Histones - form the basic tools for chromatin organization:
In eukaryotes there are five major types of Histones - H1, H2A, H2B, H3, H4.
H1 is the hypervariable histone and is least conserved. H1 is absent in yeast Saccharomyces cerevisiae. H2A and H2B are moderately conserved and H3 and H4 are highly conserved. Two copies of H2A, H2B, H3 and H4 each combine to form a histone octamer. H1 is not a part of histone octamer can be easily dissociated. Histone octamer forms the cylindrical structure around which DNA binds. H1 seals the DNA wound around the histone octamer and is therefore called linker histone. In this manner DNA is condensed by binding around the basic (positively charged) proteins – Histones, which forms the basis for chromatin organization. Packing Ratio – Levels of Chromatin Organisation: Condensation of DNA varies spatially and temporally and exhibits different .degrees of condensation which can be measured by Packing Ratio or Packing Fraction. Packing Fraction is the ratio of the actual length of the DNA (in fully extended form) to the length of the unit which contains it (condensed from). For instance, the size of the smallest human chromosome is 4.6 x 107 and is 10 times larger than the genome of E.coli and equals to 14,000 µm (1.4cm). In its most condensed form it occupies a length of 2 µm. Thus the packing ratio for chromosome is 7000. Similarly, the largest human chromosome accommodates DNA of about 500 million base pairs, occupying 17cm in length in its extended form exhibiting a packing ratio of 10,000 times. Thus, depending on the degree of condensation, there are three different levels of chromatin organization..
- 10 nm fibre
- 30 nm fibre
- Higher order structures.
As mentioned earlier histone octamer acquires a cylindrical strutcure which is 6 nm in height and 10.5 nm in width. DNA is wrapped around the cylindrical octamer by taking approximately 1.65 turns. The amount of DNA bound in the centre is more than the amount of DNA bound towards the edges. This feature of allowing more DNA to be wound in the centre leads to a phenomenon called negative supercoiling, which is highly essential for molecular mechanisms like transcription and replication. Initially, Micrococccal nuclease digestion experiments revealed approximately 200bp of DNA wound to histone octamer. But over incubation of the nuclease with the chromatin further yielded 165 bp and at last 146bp of DNA. This 146 bp of DNA is called the Core DNA and the rest of the DNA is called the linker DNA. The length of the linker DNA varies form 8-114bp. Histone octamer together with the core DNA is termed as Nucleosome which is the fundamental unit of chromatin organization. This is the primary level of chromatin organization and is also termed as the 10 nm fibre or nucleosomal model. This structure is obtained when chromatin is isolated under low ionic strength conditions and high temperature. This is more commonly known as "Beads on a string” structure and is rarely found in vivo The Packing Fraction for this level is 6.
30 nm fibre
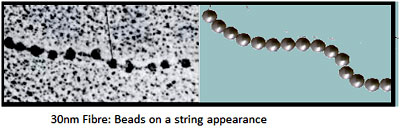
Higher order structures:
Further condensation of 30 nm fibre results in higher order structures whic exhibit diefferent states of condensation at mitotic and interphase,. At the end of mitosis, as the cells enters the interphase, the envelope reforms around the chromatin and chromosomes decondense. Some chromatin does not decondense at the end of mitosis but remains highly compacted. This densely packed chromatin displaying a condition comparable to the mitotic chromosomes is called Heterochromatin which exhibits a packing ratio of 10000 both at interphase and during mitosis. It is recognisable in interphase nuclei by light or electron microscopy and is usually located at the nuclear periphery. Heterochromatin is darkly stained and appears different from the other region and hence the name ‘hetero’ meaning ‘different’ which is primarily of two types- Constitutive and Facultative. Constitutive heterochromatin is mainly found at centromeres and telomeres, consisting primarily of highly repetitive DNA sequences. This region of chromatin always exist in heterochromatic stae and are hence considered to be permanently inactive. It also tends to repress the activity of nearby genes in a process called “Position effect or position effect variegation.
Facultative heterochromatin refers to regions of euchromatin that are converted to heterochromatin. This region contains genes, but their activity is repressed by histone methylation or small interfering RNA (siRNA). An example of facultative heterochromatin is the inactive X-chromosome in human female cells.
By contrast, euchromatin decondenses during interphase. The distinction between heterochromatin and euchromatin was originally based on different staining properties in light microscopy, but the most significant difference is that euchromatin is a transcriptionally active region as it is less condensed and contains active genes while heterochromatin does not. In the later case dislodging of histones and unfolding of DNA strands becomes a cumbersome task.
Chromosome condensation at Mitosis amd the role of Condensin and RCA:
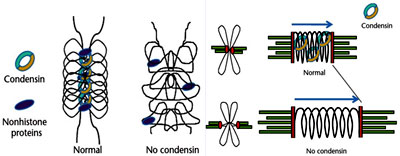
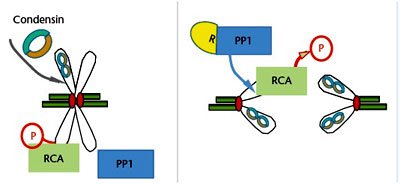
It is proposed that RCA drives chromatin condensation at mitosis and that it can do so in the absence of condensins. Its activity is sustained by Cdk1 and it is essential in prometaphase and metaphase. Inactivation of
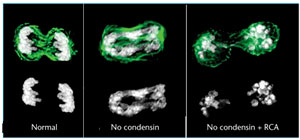
Specialised Chromosome Regions
Some regions of the chromosome appear distinct and can associated with performing specialized functions.For instance, centromere and kinetochore are involved in the proper segregation of chromatids during mitosis, and the telomeres aid in safeguarding the terminal regions of the chromosomes during DNA replication. These structures coordinate in transferring the genetic information from one generation to the other ,with high fidelity. Similarly, the nucleolar organising region (NOR) is the site of ribosomal ribonucleic acid (rRNA) synthesis and assembly of ribosomes.
Centromeres
The term centromere is named based on its location, central’ (centro) ‘region’ (mere). Walter Flemming first identified the region in 1880 as the region where the chromosome is constricted (the primary constriction). It is the region of the chromatin where the sister chromatids are held together until segregation occurs and where the kinetochore assembles providing the structure necessary for attachment of spindle microtubules. Although the name indicates that the location as central but it does not hold true in all the cases and also that not all centromeres can be represented as localised regions. For example, in the nematode Caenorhabditis elegans, the centromere is spread all along the surface of the chromosome. Despite such morphological variations, the basic structure and function of the centromere are highly conserved among species. This region is essential for the survival of cells, and alterations in its structure can cause severe problems during cell division and generate cells with abnormal chromosome number leading to aneuploidy.
The molecular nature of the centromere consists of specialized proteins that are localized to this region. Till date, there are 19 such proteins that are named as CENP for ‘CENtromeric Protein’ including the first three proteins discovered in the series, CENP-A, CENP-B and CENP-C. The presence of these proteins at the centromere can be noticed all throughout the cell cycle, and are involved in forming a network known as CCAN (Constitutive Centromere Associated Network) or CENP-A NAC/CAD (CENP-A Nucleosome Associated Complex/CENP-A Distal complex). CENP-A is the fundamental protein behind the formation of network which is a H3 variant that is found only within the centromeric chromatin. This unique organization provides a base for flexible localised chromatin appropriate for for the assembly of centromeric protein complexes,especially aid in the formation of kinetochore during mitosis. In yeast centromeres have a defined DNA sequence, whereas human centromeres are composed of a highly repetitive, divergent set of tandemly repeated sequences (satellite DNA).
The centromeric chromatin plays a very crucial biological role acting as the physical link between the replicated sister chromatids until they are separated at anaphase. Cohesion are the proteins that are mainly responsible for maintaining the link. Cohesion forms a a pentameric complex similar in composition to the condensin complex . Cohesin makes a ring that is believed to trap two DNA molecules and thereby create a physical connection between sister chromatids. At anaphase onset, the cohesin ring is cleaved by a protease called separase, and the two sister chromatids are free to separate. Another important function of centromeric chromatin is to provide rigidity to oppose the pulling forces generated by spindle microtubules attached to the sister kinetochores. Rigidity can be attributed partially to Cohesin and partially by Condensins. Absence of either of the proteins leads to loss of rigidity.
Kinetochores
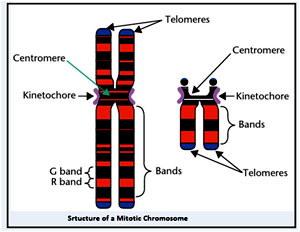
Kinetochores act as the molecular machines that not only mediate the attachment of microtubules to the sister chromatids but also verify the appropriateness of the attachments. It ensures that sister chromatids are attached to spindle microtubules emanating from opposite poles and also promotes the correction of improper attachments. In case of any corrections are needed, the anaphase is delayed until all kinetochores are correctly attached to spindle microtubules. A remarkable feature of kinetochores is that they can maintain attachment even to microtubules that are growing or disassembling.
Any error in the attachment would lead to improper segregation of sister chromatids and in order to prevent this the cell division must be stopped at that particular point. Kinetochores mediate their very essential ‘DON’T GO’ signal and is also referred to as the spindle assembly checkpoint.
Telomeres
DNA sequences present at the distal ends of chromosomes are defined as Telomeres. As in the case of eukaryotes, chromosomal DNA molecules are linear and because DNA polymerase requires an RNA primer with a 3’-hydroxyl donor group to initiate DNA replication, some nucleotides are invariably lost from the 5’ end of a DNA strand at each cell division.
To prevent this gradual loss of sequences from the ends and eventually leading to loss of important genes, telomeres are employed to prevent the process, which have a special structure and a mechanism to maintain that structure. Telomeres consist of repetitive DNA sequences, for example, in humans, hundreds to thousands of TTAGGG tandem repeats. This DNA is mostly double-stranded but has a single-stranded terminus 130–210 bases long that can fold on itself and invade the duplex telomeric DNA, resulting in the formation of a telomere loop (T loop). Telomeric DNA is also coated by a capping protein called shelterin that prevents the linear DNA molecule from being recognized by the DNA repair enzymes as a broken molecule in need of repair.
Although some repeats are inevitably lost from the 5’ end during each cycle of replication, the RNA-containing enzyme telomerase can replace them. After DNA replication has occurred, the reverse transcriptase activity of telomerase (TERT) synthesizes telomeric DNA repeats de novo using the RNA component of telomerase (TERC) as a template. When they become too short, telomeres signal the arrest of cell proliferation, senescence and apoptosis. Most somatic human tissues do not have sufficient telomerase activity to prevent telomere shortening; therefore, after several divisions, they will become senescent.
Nucleolar organising regions
NORs are clusters of ribosomal DNA (rDNA) repeats that become the sites of rRNA synthesis and ribosome assembly during interphase. In human cells, they are located on the short arms of the five acrocentric chromosomes (chromosomes 13, 14, 15, 21 and 22) in a telomere-to-centromere orientation . Most human NORs consist of approximately 70 copies of rDNA repeats and contain only rDNA. Active NORs remain undercondensed during mitosis, and they appear as secondary constrictions on metaphase chromosomes. Transcription of the genes in the regions ensures rapid resumption of ribosome biogenesis at the beginning of G1 phase.
Chromatin is present as loops and domains.
Individual chromosomes are not distinguishable in interphase nuclei. Interphase chromosomes also maintain their identity and occupy distinct ‘territories’ within the nucleus rather than becoming all ‘jumbled’ together. Although there are no internal membranes within the nucleus, but territories may be maintained by temporary attachment to filaments (nuclear matrix) that disassemble at the next mitosis. Chromatin loops anchored at their bases to the scaffold, are a feature of mitotic chromosomes. It is likely that such loops, anchored to an interphase scaffold or matrix, also persist during interphase. It has been proposed that these loops could serve as regulatory units for groups of genes, with each loop comprising of a domain in which gene expression is regulated independently of other such domains. Similar pattern is seen in the case of prokaryotes where the chromatin is attached to the plasma mebrane forming loops which act as domains.
Chromatin Remodelling:
Transcription is a process of RNA synthesis using single stranded DNA as template. Generation of single stranded DNA from double stranded requires DNA melting which is accomplished by RNA polymerase and its accessory proteins. But access to DNA for these proteins is possible only when histones bound to DNA are removed. At the same time, after accomplishment of transcription, the naked DNA must be packed again into the chromatin form to restore the original state. This dynamic behavior of chromatin to get dispensed with histones for transcription or replication and rearranging itself back into chromatin form with the assistance of various proteins, is termed as “Chromatin Remodelling”. Chromatin remodeling occurs by means of two pathways – A Replication independent pathway (occurs during transcription) and a replication coupled pathway (occurs during DNA replicatiomn). In RI pathway, reassembly of histone octamers and nucleosome formation involves incorporation of histone variants. For instance, H3.3 is a variant of H3 differing in four amino acids is preferentially incorporated into nucleosomes. This preferential bias towards H3.3 is based on its availability in the cell during interphase as in it happens in the case of the protozoan, Tetrahymena or it can be selectively picked up based on its sequence variation as in the case of Drosophila. In contrast Repilcation coupled pathway does not discriminate between H3 and H3.3 and can incorporate both but on most occasions H3.3 is available in low concentrations and therefore is represented less in the final assembly. Replication of centromeric DNA and reassembly utilizes a protein CENP-A instead of H3. Chromatin reassembly in RC pathway is linked to the replication apparatus, and requires the aid of an ancillary protein CAF-1(Chromatin assembly factor-1), which is recruited to the replication fork by another protein PCNA (Proliferating Cell Nuclear Antigen) which acts as the processivity factor for DNA polymerase.
Organization of Histones
Histone octamers have a kernel of H32-H42 tetramer associated with a dimer of H2A-H2B. The H32-H42 tetramer accounts for the diameter of the octamer and forms the core. H2A-H2B is placed on top and bottom of the tetrameric core. All the four core HIstones exhibit a characterstic structure termed as "Histone fold", with 3 alpha helices connected by two loops with the N terminal ends of all the Histones protruding outside available for different types of modifications responsible for chromatin remodeling.
Histone Modifications
The-protruding N-terminal tails, as well as some amino acids present in the carboxy-terminal part of the histones are subject to various post-translational modifications. These modifications, present individually on the nucleosome or in combination with others (constituting the histone code),together with DNA methylation as seen specifically in mammal act as determinants for the type of chromatin formed- either silent oe active.
Histone acetylation:
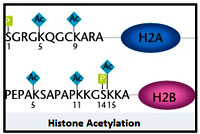
Most familiar example that histone acetylation causes transcriptional activation of genes can be witnessed in Drosophila wherein a global upregulation of genes is achieved on single X chromosomes of males so as to meet the expression levels achieved by two X chromosomes in females. Thus transcriptional activation of genes through histone acetylation is used as a mechanism to enforce Dosage Compensation. A global acetylation of lysine16 of histone H4, observed on the single X-chromosome of males is crucial to this global upregulation of transcription. In general transcriptional activation is a transient phenomenon and repression of transcription is usually established back, making Acetylation, a reversible process.Therefore deacetylation becomes compulsory and is executed by different classes of histone deacetylases (HDACs).Alterations in the histone acetylation plays a key role in normal cell growth is dependent on the dynamic interplay of HATs and HDACs. A number of inhibitors of HDACs are currently used as anticancer agents and others are under clinical evaluation.
Histone methylation
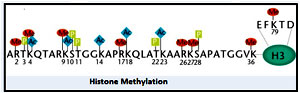
Type I enzymes catalyze asymmetrical dimethyl arginine and type II enzymes generate symmetrical dimethyl arginine. The potential functional activity of arginine methylation depends on the residue being modified and the type of methylation introduced. Symmetrical and asymmetrical methylation of the arginine3 on histone H4 exhibit opposite effects on transcription regulation. Indeed, whereas PRMT1-mediated asymmetrical methylation correlates with transcriptional activation through a specific interplay with histone acetylation,PRMT5-mediated symmetrical methylation is linked to transcriptional repression. Similar to acetylation, arginine methylation also needs to be dynamically regulated. Two types of enzymes are responsible for demethyaltion on arginine- PAD-4 and JMJD6.
Apart from arginine methylation, histone methyl transferases cause different degrees of lysine methylation (mono-,di-,tri-) that strongly potentialize the cellular interpretation of each lysine methylation mark. By and large methylation leads to condensation of chromatin but depending on the degree of methylation, methylation marks, even on the same histone, could activate transcription or in contrast, participate in the formation of condensed structures. For instance trimethylation of the lysine4 on histone H3 (H3K4me3) is essential for transcriptional activation,whereas trimethylation of lysine9 or of lysine 27on H3 (H3K9me3, H3K27me3) favours the chromatin condensation. In the latter case,H3K9me3 and H3K27me3 marks attract the structural chromodomain-containing proteins HP1and polycomb (PC) responsible for the formation of constitutive and facultative heterochromatin, respectively. Until recently, methylation of lysines was considered to be an irreversible mark but with the discovery of specific lysine demethylases like LSD1, has been now demonstrated that lysine methylation can be dynamically regulated.
Histone phosphorylation:
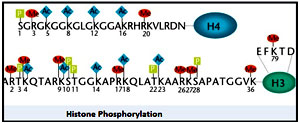
Histone ubiquitination
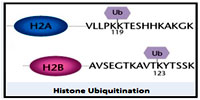
Published date : 19 Jun 2014 01:48PM