Membrane Transport
Sakshi Education
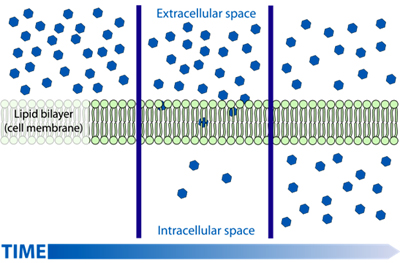
Passive transport is a means of moving biochemicals, and other atomic or molecular substances, across membranes. Unlike active transport, this process does not involve chemical energy. Passive transport is dependent on the permeability of the cell membrane, which, in turn, is dependent on the organization and characteristics of the membrane lipids and proteins. The four main kinds of passive transport are diffusion, facilitated diffusion, filtration and osmosis
Diffusion:
Diffusion is the net movement of material from an area of high concentration of that material to an area with lower concentration. The difference of concentration between the two areas is often termed as the concentration gradient, and diffusion will continue until this gradient has been eliminated. Since diffusion moves materials from an area of higher concentration to the lower, it is described as moving solutes "down the concentration gradient" (compared with active transport, which often moves material from area of low concentration to area of higher concentration, and therefore referred to as moving the material "against the concentration gradient").
Facilitated Diffusion:
Facilitated diffusion is movement of molecules across the cell membrane via special transport proteins that are embedded within the cellular membrane. Many large molecules, such as glucose, are insoluble in lipids and too large to fit through the membrane pores. Therefore, it will bind with its specific carrier proteins, and the complex will then be bonded to a receptor site and moved through the cellular membrane. Bear in mind, however, that facilitated diffusion is a passive process, and the solutes still move down the concentration gradient. The alveoli are tiny grapelike sacs located at the end of the bronchial tubes. This is where oxygen diffuses into the alveoli and is exchanged for carbon dioxide.
Ex: Na+ - Glucose Transport
Filteration:
Filtration is movement of water and solute molecules across the cell membrane due to hydrostatic pressure generated by the cardiovascular system. Depending on the size of the membrane pores, only solutes of a certain size may pass through it. For example, the membrane pores of the Bowman's capsule in the kidneys are very small, and only albumins, the smallest of the proteins, have any chance of being filtered through. On the other hand, the membrane pores of liver cells are extremely large, to allow a variety of solutes to pass through and be metabolized.
Osmosis:
Osmosis is the net movement of a solvent across a semipermeable membrane from a region of high solvent potential to an area of low solvent potential down a concentration gradient. It is a physical process in which a solvent moves, without input of energy, across a semipermeable membrane (permeable to the solvent, but not the solute) separating two solutions of different concentrations. Osmosis releases energy, and can be made to do work, as when a growing tree-root splits a stone.
Osmotic pressure is the main cause of support in many plants. The osmotic entry of water raises the turgor pressure exerted against the cell wall, until it equals the osmotic pressure, creating a steady state.
When a plant cell is placed in a hypertonic solution, the water in the cells moves to an area higher in solute concentration, and the cell shrinks and so becomes flaccid [pron. flassid]. (This means the cell has become plasmolysed - the cell membrane has completely left the cell wall due to lack of water pressure on it; the opposite of turgid.)
Also, osmosis is responsible for the ability of plant roots to suck up water from the soil. Since there are many fine roots, they have a large surface area, water enters the roots by osmosis, and generates the pressure required for the water to travel up the plant.
Osmosis can also be seen very effectively when potato slices are added to a high concentration of salt solution. The water from inside the potato moves to the salt solution, causing the potato to shrink and to lose its 'turgor pressure'. The more concentrated the salt solution, the bigger the difference in size and weight of the potato slice.
In unusual environments, osmosis can be very harmful to organisms. For example, if freshwater and saltwater aquarium fishes placed in water of a different salinity (than they are adapted to) will die quickly, and in the case of saltwater fish rather dramatically. Additionally, note the use of table salt to kill leeches and slugs.
Suppose we place an animal or a plant cell in a solution of sugar or salt in water.
If the medium is hypotonic — a dilute solution, with a higher water concentration than the cell — the cell will gain water through osmosis.
If the medium is isotonic — a solution with exactly the same water concentration as the cell — there will be no net movement of water across the cell membrane.
If the medium is hypertonic — a concentrated solution, with a lower water concentration than the cell — the cell will lose water by osmosis.
Plasmolysis - is the term which describes plant cells when the cytoplasm shrinks from the cell wall in a hypertonic environment. In plasmolysis, the cell wall stays in tact, but the plasma mebrane shrinks and the chloroplasts of the plant cell concentrate in the center of the cell.
Cytolysis - is the term which describes the bursting of cells without cell walls in a hypotonic environment.
Hemolysis - If a red blood cell were exposed to pure water with a solute concentration of zero, the diffusion of the water or osmosis would diffuse from a concentration fo high to low. The water would then be considered a hypotonic solution and the water would then enter the red blood cell. But many times, too much water enters the red blood cell and results in the bursting of the red blood cell, otherwise known as hemolysis. Hemolysis gets its name from the prefix hemo- which means blood and the suffix -lysis which means destruction or dissolution.
Crenation is the contraction or formation of abnormal notchings around the edges of a cell after exposure to a hypertonic solution, due to the loss of water through osmosis. The word is from the Latin "crenatus" meaning scalloped or notched, and is named for the scalloped-edged shape the cells take on when crenated.
Reverse Osmosis:
The osmosis process can be driven in reverse, with solvent moving from a region of high solute concentration to a region of low solute concentration by applying a pressure in excess of the osmotic pressure. Recent advances in pressure exchange and the ongoing development of low pressure membranes have significantly reduced the costs of water purified by reverse osmosis. The reverse osmosis technique is commonly applied in desalination, water purification, water treatment, and food processing.
Forward Osmosis:
Osmosis may be used directly to achieve separation of water from a "feed" solution containing unwanted solutes. A "draw" solution of higher osmotic pressure than the feed solution is used to induce a net flow of water through a semi-permeable membrane, such that the feed solution becomes concentrated as the draw solution becomes dilute. The diluted draw solution may then be used directly (as with an ingestible solute like glucose), or sent to a secondary separation process for the removal of the draw solute. This secondary separation can be more efficient than a reverse osmosis process would be alone, depending on the draw solute used and the feedwater treated. Forward osmosis is an area of ongoing research, focusing on applications in desalination, water purification, water treatment, and food processing.
Active transport (sometimes called active uptake) is the mediated transport of biochemicals, and other atomic/molecular substances, across membranes. Unlike passive transport, this process requires the expenditure of cellular energy to move molecules "uphill" against a gradient.
Types
In primary transport, energy from hydrolysis of ATP is directly coupled to the movement of a specific substance across a membrane independent of any other species.
In secondary active transport, the required energy is derived from energy stored in the form of concentration differences in a second solute. Typically, the concentration gradient of the second solute was created by primary active transport, and the diffusion of the second solute across the membrane drives secondary active transport. In secondary active transport, in contrast to primary active transport, there is no direct coupling of ATP; instead, the electrochemical potential difference created by pumping ions out of the cell is used.
The three main forms of this are uniport, counter-transport (antiport) and co-transport (symport).
A uniporter is an integral membrane protein that is involved in facilitated diffusion. They can be either a channel or a carrier protein.
Uniporter carrier proteins work by binding to one molecule of solute at a time and transporting it with the solute gradient. Uniporter channels open in response to a stimulus and allow the free flow of specific molecules. Uniporters may not utilize energy other than the solute gradient. Thus they may only transport molecules with the solute gradient, and not against it.
There are several ways in which the opening of uniporter channels may be regulated:
Diffusion:
Diffusion is the net movement of material from an area of high concentration of that material to an area with lower concentration. The difference of concentration between the two areas is often termed as the concentration gradient, and diffusion will continue until this gradient has been eliminated. Since diffusion moves materials from an area of higher concentration to the lower, it is described as moving solutes "down the concentration gradient" (compared with active transport, which often moves material from area of low concentration to area of higher concentration, and therefore referred to as moving the material "against the concentration gradient").
Facilitated Diffusion:
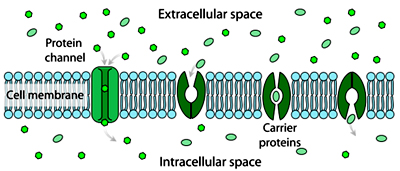
Ex: Na+ - Glucose Transport
Filteration:
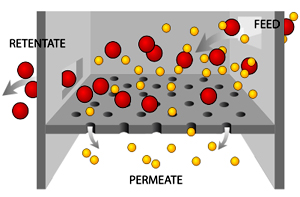
Osmosis:
Osmosis is the net movement of a solvent across a semipermeable membrane from a region of high solvent potential to an area of low solvent potential down a concentration gradient. It is a physical process in which a solvent moves, without input of energy, across a semipermeable membrane (permeable to the solvent, but not the solute) separating two solutions of different concentrations. Osmosis releases energy, and can be made to do work, as when a growing tree-root splits a stone.
Osmotic pressure is the main cause of support in many plants. The osmotic entry of water raises the turgor pressure exerted against the cell wall, until it equals the osmotic pressure, creating a steady state.
When a plant cell is placed in a hypertonic solution, the water in the cells moves to an area higher in solute concentration, and the cell shrinks and so becomes flaccid [pron. flassid]. (This means the cell has become plasmolysed - the cell membrane has completely left the cell wall due to lack of water pressure on it; the opposite of turgid.)
Also, osmosis is responsible for the ability of plant roots to suck up water from the soil. Since there are many fine roots, they have a large surface area, water enters the roots by osmosis, and generates the pressure required for the water to travel up the plant.
Osmosis can also be seen very effectively when potato slices are added to a high concentration of salt solution. The water from inside the potato moves to the salt solution, causing the potato to shrink and to lose its 'turgor pressure'. The more concentrated the salt solution, the bigger the difference in size and weight of the potato slice.
In unusual environments, osmosis can be very harmful to organisms. For example, if freshwater and saltwater aquarium fishes placed in water of a different salinity (than they are adapted to) will die quickly, and in the case of saltwater fish rather dramatically. Additionally, note the use of table salt to kill leeches and slugs.
Suppose we place an animal or a plant cell in a solution of sugar or salt in water.
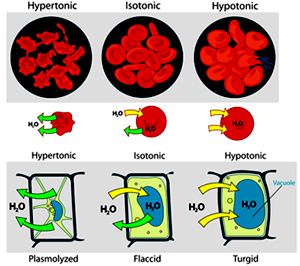
If the medium is isotonic — a solution with exactly the same water concentration as the cell — there will be no net movement of water across the cell membrane.
If the medium is hypertonic — a concentrated solution, with a lower water concentration than the cell — the cell will lose water by osmosis.
Plasmolysis - is the term which describes plant cells when the cytoplasm shrinks from the cell wall in a hypertonic environment. In plasmolysis, the cell wall stays in tact, but the plasma mebrane shrinks and the chloroplasts of the plant cell concentrate in the center of the cell.
Cytolysis - is the term which describes the bursting of cells without cell walls in a hypotonic environment.
Hemolysis - If a red blood cell were exposed to pure water with a solute concentration of zero, the diffusion of the water or osmosis would diffuse from a concentration fo high to low. The water would then be considered a hypotonic solution and the water would then enter the red blood cell. But many times, too much water enters the red blood cell and results in the bursting of the red blood cell, otherwise known as hemolysis. Hemolysis gets its name from the prefix hemo- which means blood and the suffix -lysis which means destruction or dissolution.
Crenation is the contraction or formation of abnormal notchings around the edges of a cell after exposure to a hypertonic solution, due to the loss of water through osmosis. The word is from the Latin "crenatus" meaning scalloped or notched, and is named for the scalloped-edged shape the cells take on when crenated.
Reverse Osmosis:
The osmosis process can be driven in reverse, with solvent moving from a region of high solute concentration to a region of low solute concentration by applying a pressure in excess of the osmotic pressure. Recent advances in pressure exchange and the ongoing development of low pressure membranes have significantly reduced the costs of water purified by reverse osmosis. The reverse osmosis technique is commonly applied in desalination, water purification, water treatment, and food processing.
Forward Osmosis:
Osmosis may be used directly to achieve separation of water from a "feed" solution containing unwanted solutes. A "draw" solution of higher osmotic pressure than the feed solution is used to induce a net flow of water through a semi-permeable membrane, such that the feed solution becomes concentrated as the draw solution becomes dilute. The diluted draw solution may then be used directly (as with an ingestible solute like glucose), or sent to a secondary separation process for the removal of the draw solute. This secondary separation can be more efficient than a reverse osmosis process would be alone, depending on the draw solute used and the feedwater treated. Forward osmosis is an area of ongoing research, focusing on applications in desalination, water purification, water treatment, and food processing.
Active transport (sometimes called active uptake) is the mediated transport of biochemicals, and other atomic/molecular substances, across membranes. Unlike passive transport, this process requires the expenditure of cellular energy to move molecules "uphill" against a gradient.
Types
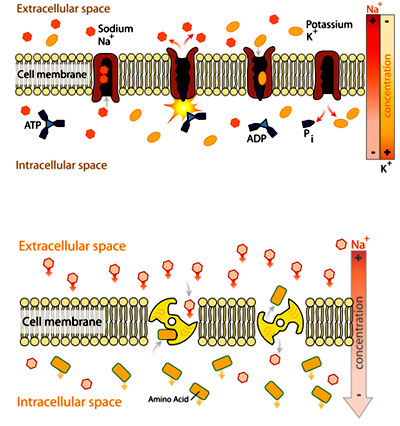
In secondary active transport, the required energy is derived from energy stored in the form of concentration differences in a second solute. Typically, the concentration gradient of the second solute was created by primary active transport, and the diffusion of the second solute across the membrane drives secondary active transport. In secondary active transport, in contrast to primary active transport, there is no direct coupling of ATP; instead, the electrochemical potential difference created by pumping ions out of the cell is used.
The three main forms of this are uniport, counter-transport (antiport) and co-transport (symport).
A uniporter is an integral membrane protein that is involved in facilitated diffusion. They can be either a channel or a carrier protein.
Uniporter carrier proteins work by binding to one molecule of solute at a time and transporting it with the solute gradient. Uniporter channels open in response to a stimulus and allow the free flow of specific molecules. Uniporters may not utilize energy other than the solute gradient. Thus they may only transport molecules with the solute gradient, and not against it.
There are several ways in which the opening of uniporter channels may be regulated:
- Voltage - Regulated by the difference in voltage across the membrane
- Stress - Regulated by physical pressure on the transporter (as in the cochlea of the ear)
- Ligand - Regulated by the binding of a ligand to either the intracellular or extracellular side of the cell
In the ear, sound waves cause the stress-regulated channels in the ear to open, sending an impulse to the vestibulocochlear nerve (VIII).
Diseases of Ion Channels
There are a number of chemicals and genetic disorders which disrupt normal functioning of ion channels and have disastrous consequences for the organism. Genetic disorders of ion channels and their modifiers are known as Channelopathies. See Category:Channelopathy for a full list.
Chemicals
- Tetrodotoxin (TTX), used by puffer fish and some types of newts for defense. It is a sodium channel blocker.
- Saxitoxin, produced by a dinoflagellate also known as red tide. It blocks voltage dependent sodium channels.
- Conotoxin, which is used by cone snails to hunt prey.
- Lidocaine andNovocaine belong to a class of local anesthetics which block sodium ion channels.
- Dendrotoxin is produced by mamba snakes which blocks potassium channels.
- Shaker gene mutations cause a defect in the voltage gated ion channels, slowing down the repolarization of the cell.
- Equine hyperkalaemic periodic paralysis as well as Human hyperkalaemic periodic paralysis (HyperPP) are caused by a defect in voltage dependent sodium channels.
- Paramyotonia congenita (PC) and potassium aggravated myotonias (PAM)
- Generalized epilepsy with febrile seizures plus (GEFS+)
- Episodic Ataxia (EA)
- Familial hemiplegic migraine (FHM)
- spinocerebellar ataxia type 13
- Long QT syndrome is a ventricular arrhythmia syndrome caused by mutations in one or more of presently ten different genes, most of which are potassium channels and all of which affect cardiac repolarization.
- Brugada syndrome is another ventricular arrhythmia caused by voltage-gated sodium channel gene mutations.
- Cystic fibrosis is caused by mutations in the CFTR gene, which is a chloride channel.
In counter-transport two species of ion or other solutes are pumped in opposite directions across a membrane. One of these species is allowed to flow from high to low concentration which yields the entropic energy to drive the transport of the other solute from a low concentration region to a high one. An example is the sodium-calcium exchanger or antiporter, which allows three sodium ions into the cell to transport one calcium out.
Many cells also possess a calcium ATPase, which can operate at lower intracellular concentrations of calcium and sets the normal or resting concentration of this important second messenger. But the ATPase exports calcium ions more slowly: only 30 per second versus 2000 per second by the exchanger. The exchanger comes into service when the calcium concentration rises steeply or "spikes" and enables rapid recovery. This shows that a single type of ion can be transported by several enzymes, which need not be active all the time (constitutively), but may exist to meet specific, intermittent needs.
Co-transport
Co-transport uses the downhill movement of one solute species from high to low concentration to move another molecule uphill from low concentration to high concentration (against its electrochemical gradient).
An example is the glucose symporter SGLT1, which co-transports one glucose (or galactose) molecule into the cell for every two sodium ions it imports into the cell. This symporter is located in the small intestines, trachea, heart, brain, testis, and prostate. It is also located in the S3 segment of the proximal tubule in each nephron in the kidneys. Its mechanism is exploited in glucose rehydration therapy and defects in SGLT1 prevent effective reabsorption of glucose, causing familial renal glucosuria.
Published date : 10 May 2014 03:43PM