Cell Junctions
Sakshi Education

Interactions of cells of same kind with each other (e.g. the endothelial cells lining the blood vessels) are called Homotypic interactions. However cells communicating among themselves is not one and the same, that act is called as Heterotypic interaction (e.g. cells cooperating during the immune response). Cell-to-cell junctions (In short - cell junctions) are needed for cellular interactions.
Based on the functions they perform, cell junctions are divided into three categories: (1) those that tie adjacent cells together mechanically; (2) those that allow for communication or metabolic coupling between adjacent cells; and (3) those that seal the extracellular cleft between adjacent cells so that the diffusion of solutes through the intercellular space is restricted.
Tight Junctions (TJ): Barrier-Forming Cell-to-Cell Contacts
The TJ (the former Latin term is Zonula Occludens or ZO) are localised and very close to the apical pole of the cells at the entrance of the intercellular cleft. These cell-cell contacts are the boundary that separates the apical from the basolateral membrane domain. Two basic functions are assigned to the TJ are: the ‘gate’ and ‘fence’ functions.
The gate function describes the tight occlusion of the intercellular cleft so that solutes cannot simply diffuse from the apical to the basolateral compartment or vice versa. Essentially they act as barriers, for instance they restrict the entry of polar compounds across the plasma membrane of epithelial cell. Only few selected molecules having suitable transposrt proteins can be transported inside the cell. Thus they exhibit high specificity and also allow for the establishment of chemical or electrochemical gradients across the epithelial cell layer essential for strict regulation of uptake of metabolites like glucose.
TJ also perform fence function, preserving the membrane asymmetry and polarity by preventing the movement of proteins between both membrane domains, which is also essential for transepithelial transport. Thus TJ restrict diffusion through the intercellular cleft and also within the cellular plasma membrane. Once proteins have been integrated either in the apical or the basolateral membrane domain by the protein targeting machinery, they cannot diffuse through the TJ and migrate into the other part of the membrane.
TJ can be identified as a 0.1–0.7mm wide belt in which the opposing plasma membranes form intimate contacts that resemble points of membrane fusion and are thus called membrane kisses. At these membrane kisses the intercellular diffusion pathway is effectively blocked or occluded by means of a network of two-dimensional strands that surrounds the cell like a belt. The number of strands and the complexity of the network determine the junctional tightness. A whole family of proteins, the claudin family forms the major constituent of the tight junction strands.
Examples of the most prominent tight junction-specific or tight junction-associated proteins are occludin, junctional adhesion molecule (JAM), tricellulin, ZO-1, ZO-2, CAR, ESAM.
The claudins, occludin and also tricellulin are very similar with respect to their three-dimensional topology, although they have very little sequence similarity. They have four transmembrane domains, which form two closed extracellular loops with the N- and C-termini of the protein both located in the cytoplasm. The two extracellular loops from opposing cells are thought to interact and form the actual seal of the intercellular cleft. It is, however, well known that some members of the claudin family build size- and cation specific pores into the TJ strands, which explains their individual barrier properties towards different tracer compounds. TJ are usually considered as being robust and static structures but can be highly dynamic and can adjust their barrier properties. Some of the tight junction-specific transmembrane proteins are connected to the actin cytoskeleton via several linker or adapter proteins, so that the total molecular architecture of TJ closely resembles that of Adherens Junctions and Desmosomes.
Adherens junctions (AJ)
Adherens junctions (former Latin term is Zonula Adhaerens or ZA) or AJ can be found right underneath TJ, followed by the Desmosomes (DS) further down moving along the intercellular cleft between two epithelial cells in apical to basolateral direction. AJ surround the cells like a belt connected to thick bundles of actin filaments close to the apical surface of the cell and were thus previously called Belt desmosomes. Whereas the DS are more spot like structures distributed along the contact area between adjacent cells and linked to intermediate filaments that traverse the entire cytoplasm and form a dense network around the nucleus.
Like typical transmembrane junctional proteins, AJ and DS make contact with corresponding transmembrane protein on the opposing cell surface via their extracellular domains, whereas their intracellular domains are connected to the cytoskeleton via an individual set of adapter proteins. Accordingly, these two cell junctions interconnect both the microfilaments and the intermediate filaments of individual cells so that they run – in a sense continuously – through the whole epithelial monolayer. The mechanical interconnection of the filaments that is provided by AJ and DS gives epithelial cell layers a very unique mechanical stability, which is most obvious in skin epithelium. It is, for instance, a daily experience that the skin does not show cuts and openings even for mechanical load forces that would be too severe. Members of the cadherin protein family are the exclusive transmembrane component in DS and one out of two types of transmembrane proteins in AJ.
Cadherins were named according to their Ca2+-dependent adhesion functionality. Cadherins found in AJ are termed classical cadherins compared to desmosomal cadherins in DS, which are further subdivided into desmogleins and desmocollins. Cadherins in general form single-span transmembrane proteins with five extracellular domains and a total of four Ca2+-binding sites at the interfaces between the five extracellular domains. Cadherins expressed on the same cell surface form so-called Cis-dimers, which then establish homophilic interactions with cis-dimers on the opposing cell surface. These constructs are then referred to as transdimers. In mature AJ a large number of cadherin dimers group together and, because of their interdigitated and alternating association, the resulting structures are called cadherin zippers forming the belt-like AJ. This model of cadherin interactions is also proposed for DS. One major difference between cadherins found in AJ and those present in DS is their individual cytoplasmic domain. The intracellular parts of the proteins determine which adapter proteins can bind and, thus, which kind of cytosekeletal filament is connected to the transmembrane components of the junction. The most prominent linker proteins in AJ are ß- and p120-catenins that directly bind to the cytoplasmic domain reffered as classical cadherins.
Besides cadherins there is a second class of transmembrane proteins that is involved in adherens junction formation, the nectins. Nectins are calcium-independent cell adhesion molecules with one single transmembrane domain and they belong to the immunoglobulin superfamily.
Cell adhesion outside mature junctions
More about Cadherins
Cadherins are categorised by the tissue in which they are predominantly expressed. E-cadherin received its name from its prominent occurrence on epithelial cells, whereas VE-cadherin is assigned to vascular endothelial cells. N-cadherin is found on nerve and heart cells, whereas P-cadherin is characteristic for cells derived from the placenta and the epidermis.
There is also strong evidence that the metastatic potential of carcinoma cells (i.e. tumour cells that have developed from epithelial cells) is strongly correlated with the expression of E-cadherin. Loss of E-cadherin-mediated cell–cell interactions allows carcinoma cells to leave the primary tumour and migrate through the adjacent tissue into the blood vessels and beyond.
Other cell adhesion molecules
In addition to cadherins, other proteins have been assigned to the group of cell adhesion molecules (CAMs) which similar to nectins predominantly mediate Ca2+ independent cell adhesion. The neural cell adhesion molecule (NCAM), highly expressed on nerve cells, is the best characterized molecule.
The intercellular adhesion molecule (ICAM) is expressed on stimulated endothelial cells and it interacts with a certain subtype of surface proteins on leucocytes, the integrins. This heterotypic interaction allows the leucocytes to bind to the vessel wall and extravasate into the surrounding tissue. A very similar function is ascribed to a group of proteins referred to as selectins, which are also expressed on endothelial cells. These proteins recognise certain carbohydrate structures on the surface of leucocytes and thereby form a site of heterotypic cell adhesion.
Plasmodesmata: Communicating Cell Junctions
Synapses, Gap junctions and Plasmodesmata share a basic function, serving as cell junctions for communication purposes.
Synapses
Synapses are very special cell junctions between two neurons (interneuronal junctions) or neurons and muscle cells (neuromuscular junctions). In these junctions, the membrane of the so-called presynaptic cell, which sends a signal, is locally brought into very close contact with the membrane of the postsynaptic cell, which receives and processes the signal. The synaptic cleft between these two membranes is only 20– 30 nm wide and it is filled with components of the extracellular matrix (ECM). This protein and glycoprotein filling is one mechanism by which the membranes of the two cells are locally stabilised in such close apposition. Both cells on either side of the junction express the corresponding cell surface receptors, so the ECM serves as an adhesion mediator or molecular glue. A molecular assembly that is ultrastructurally very similar to AJ in epithelial cells, called asynaptic adherens junction, also provides mechanical stability at synapses. N-cadherin transmembrane proteins on the surface of either cell form clustered homophilic interactions in the synaptic cleft which are stabilised by their linkage to the microfilament system via a set of intracellular adapter proteins. An action potential arriving at the presynaptic membrane triggers the fusion of neurotransmitter-loaded vesicles with the axonal plasma membrane. The neurotransmitters are released into the synaptic cleft, diffuse very fast across this narrow extracellular space and bind to corresponding transmitter-gated ion channels on the postsynaptic membrane. Upon channel opening, the associated influx of ions depolarises the cell and thereby generates an action potential that can now migrate along the membrane of the postsynaptic nerve or muscle cell. Any excess of the neurotransmitter in the synaptic cleft that is no longer needed is quickly removed and the state of the synaptic junction is regenerated. Taken together, synaptic junctions provide the molecular requirements to transmit electrical impulses from one cell to the next via a burst of a transmitter molecule secreted into a defined volume close to the receiver membrane where the corresponding receptors are located.
Gap Junctions (GJ) and Plasmodesmata:
By a very different mechanism both GJ and plasmodesmata allow the communication between two adjacent GJ, also referred to as electrical synapses, are basically formed by a group of transmembrane proteins called connexins. Six of these connexins are arranged in such a way that a hydrophilic pore opens up in the core of the hexagon. This functional unit of a gap junction is named a connexon. When this connexon hemichannel binds a corresponding structure on the surface of the opposite cell, a direct, water filled connection of approximately 1.5 nm diameter is formed between the two cytoplasms. These channels can be used to share information and also to allow diffusion of metabolites from one cell to the other (metabolic coupling). GJ are, however, size-selective. Molecules up to a molecular weight of 1000 g mol -1 (1 kDa) such as sugars, amino acids and nucleotides, can almost freely diffuse through the pore whereas larger molecules are rejected.
Accordingly, the cells may share small metabolites and ions but not macromolecules like proteins, deoxyribonucleic acid or ribonucleic acid. The term electrical synapses, in contrast to the chemical synapses described in the preceding paragraph, arose because GJ allow a free flow of ions from one cell to the next through the gap junction channels, which provides the requirements to transmit electrical signals without making use of chemical transmitters. This mode of action potential propagation is faster than the transmitter-based mechanism described above in neural synapses. Heart muscle cells are extensively interconnected by GJ, which provides the basis for highly synchronized contraction of the heart muscle. The participating cells can adjust the permeability of the gap junction channels. When the connexon hemichannels are open, the six connexin proteins are somewhat tilted with respect to the direction of the channel. A sudden increase of the intracellular Ca2+ concentration or a drop of pH provides conformational changes within the connexins, which eventually change their tilt angles and thereby close the channel. This is a rather important safety precaution because it avoids that breakdown of the osmotic balance in one cell, for instance when the plasma membrane has become permeable for any reason, propagates into all neighboring cells and destroys the tissue.
Plasmodesmata between plant cells have a very similar function, although the structure is rather different. This different structural solution for the same problem is apparently necessary because the rather thick cell wall does not allow plant cells to get into sufficiently intimate contact to build gap junction-like structures in which proteins bridge between the two cytoplasms. At plasmodesmata, the otherwise continuous cell wall around plant cells forms point-like openings at which the plasma membranes of neighbouring cells fuse. This provides a continuous channel lined by the plasma membrane, which is roughly 30–50 nm in diameter (compared to 1.5 nm for gap junction channels). To reduce the size of this connection and to provide some size selectivity, a membrane vesicle (desmotubule) that has budded off the endoplasmic reticulum acts like a cork in a bottle. This plugging of the intercellular connection slows down diffusional exchange and only allows sufficiently small molecules to migrate into neighbouring cells.
References
Based on the functions they perform, cell junctions are divided into three categories: (1) those that tie adjacent cells together mechanically; (2) those that allow for communication or metabolic coupling between adjacent cells; and (3) those that seal the extracellular cleft between adjacent cells so that the diffusion of solutes through the intercellular space is restricted.
Tight Junctions (TJ): Barrier-Forming Cell-to-Cell Contacts
The TJ (the former Latin term is Zonula Occludens or ZO) are localised and very close to the apical pole of the cells at the entrance of the intercellular cleft. These cell-cell contacts are the boundary that separates the apical from the basolateral membrane domain. Two basic functions are assigned to the TJ are: the ‘gate’ and ‘fence’ functions.
The gate function describes the tight occlusion of the intercellular cleft so that solutes cannot simply diffuse from the apical to the basolateral compartment or vice versa. Essentially they act as barriers, for instance they restrict the entry of polar compounds across the plasma membrane of epithelial cell. Only few selected molecules having suitable transposrt proteins can be transported inside the cell. Thus they exhibit high specificity and also allow for the establishment of chemical or electrochemical gradients across the epithelial cell layer essential for strict regulation of uptake of metabolites like glucose.
TJ also perform fence function, preserving the membrane asymmetry and polarity by preventing the movement of proteins between both membrane domains, which is also essential for transepithelial transport. Thus TJ restrict diffusion through the intercellular cleft and also within the cellular plasma membrane. Once proteins have been integrated either in the apical or the basolateral membrane domain by the protein targeting machinery, they cannot diffuse through the TJ and migrate into the other part of the membrane.
TJ can be identified as a 0.1–0.7mm wide belt in which the opposing plasma membranes form intimate contacts that resemble points of membrane fusion and are thus called membrane kisses. At these membrane kisses the intercellular diffusion pathway is effectively blocked or occluded by means of a network of two-dimensional strands that surrounds the cell like a belt. The number of strands and the complexity of the network determine the junctional tightness. A whole family of proteins, the claudin family forms the major constituent of the tight junction strands.
Examples of the most prominent tight junction-specific or tight junction-associated proteins are occludin, junctional adhesion molecule (JAM), tricellulin, ZO-1, ZO-2, CAR, ESAM.
The claudins, occludin and also tricellulin are very similar with respect to their three-dimensional topology, although they have very little sequence similarity. They have four transmembrane domains, which form two closed extracellular loops with the N- and C-termini of the protein both located in the cytoplasm. The two extracellular loops from opposing cells are thought to interact and form the actual seal of the intercellular cleft. It is, however, well known that some members of the claudin family build size- and cation specific pores into the TJ strands, which explains their individual barrier properties towards different tracer compounds. TJ are usually considered as being robust and static structures but can be highly dynamic and can adjust their barrier properties. Some of the tight junction-specific transmembrane proteins are connected to the actin cytoskeleton via several linker or adapter proteins, so that the total molecular architecture of TJ closely resembles that of Adherens Junctions and Desmosomes.
Adherens junctions (AJ)
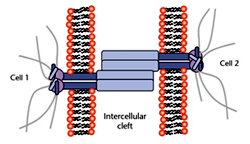
Like typical transmembrane junctional proteins, AJ and DS make contact with corresponding transmembrane protein on the opposing cell surface via their extracellular domains, whereas their intracellular domains are connected to the cytoskeleton via an individual set of adapter proteins. Accordingly, these two cell junctions interconnect both the microfilaments and the intermediate filaments of individual cells so that they run – in a sense continuously – through the whole epithelial monolayer. The mechanical interconnection of the filaments that is provided by AJ and DS gives epithelial cell layers a very unique mechanical stability, which is most obvious in skin epithelium. It is, for instance, a daily experience that the skin does not show cuts and openings even for mechanical load forces that would be too severe. Members of the cadherin protein family are the exclusive transmembrane component in DS and one out of two types of transmembrane proteins in AJ.
Cadherins were named according to their Ca2+-dependent adhesion functionality. Cadherins found in AJ are termed classical cadherins compared to desmosomal cadherins in DS, which are further subdivided into desmogleins and desmocollins. Cadherins in general form single-span transmembrane proteins with five extracellular domains and a total of four Ca2+-binding sites at the interfaces between the five extracellular domains. Cadherins expressed on the same cell surface form so-called Cis-dimers, which then establish homophilic interactions with cis-dimers on the opposing cell surface. These constructs are then referred to as transdimers. In mature AJ a large number of cadherin dimers group together and, because of their interdigitated and alternating association, the resulting structures are called cadherin zippers forming the belt-like AJ. This model of cadherin interactions is also proposed for DS. One major difference between cadherins found in AJ and those present in DS is their individual cytoplasmic domain. The intracellular parts of the proteins determine which adapter proteins can bind and, thus, which kind of cytosekeletal filament is connected to the transmembrane components of the junction. The most prominent linker proteins in AJ are ß- and p120-catenins that directly bind to the cytoplasmic domain reffered as classical cadherins.
Besides cadherins there is a second class of transmembrane proteins that is involved in adherens junction formation, the nectins. Nectins are calcium-independent cell adhesion molecules with one single transmembrane domain and they belong to the immunoglobulin superfamily.
Cell adhesion outside mature junctions
More about Cadherins
Cadherins are categorised by the tissue in which they are predominantly expressed. E-cadherin received its name from its prominent occurrence on epithelial cells, whereas VE-cadherin is assigned to vascular endothelial cells. N-cadherin is found on nerve and heart cells, whereas P-cadherin is characteristic for cells derived from the placenta and the epidermis.
There is also strong evidence that the metastatic potential of carcinoma cells (i.e. tumour cells that have developed from epithelial cells) is strongly correlated with the expression of E-cadherin. Loss of E-cadherin-mediated cell–cell interactions allows carcinoma cells to leave the primary tumour and migrate through the adjacent tissue into the blood vessels and beyond.
Other cell adhesion molecules
In addition to cadherins, other proteins have been assigned to the group of cell adhesion molecules (CAMs) which similar to nectins predominantly mediate Ca2+ independent cell adhesion. The neural cell adhesion molecule (NCAM), highly expressed on nerve cells, is the best characterized molecule.
The intercellular adhesion molecule (ICAM) is expressed on stimulated endothelial cells and it interacts with a certain subtype of surface proteins on leucocytes, the integrins. This heterotypic interaction allows the leucocytes to bind to the vessel wall and extravasate into the surrounding tissue. A very similar function is ascribed to a group of proteins referred to as selectins, which are also expressed on endothelial cells. These proteins recognise certain carbohydrate structures on the surface of leucocytes and thereby form a site of heterotypic cell adhesion.
Plasmodesmata: Communicating Cell Junctions
Synapses, Gap junctions and Plasmodesmata share a basic function, serving as cell junctions for communication purposes.
Synapses
Synapses are very special cell junctions between two neurons (interneuronal junctions) or neurons and muscle cells (neuromuscular junctions). In these junctions, the membrane of the so-called presynaptic cell, which sends a signal, is locally brought into very close contact with the membrane of the postsynaptic cell, which receives and processes the signal. The synaptic cleft between these two membranes is only 20– 30 nm wide and it is filled with components of the extracellular matrix (ECM). This protein and glycoprotein filling is one mechanism by which the membranes of the two cells are locally stabilised in such close apposition. Both cells on either side of the junction express the corresponding cell surface receptors, so the ECM serves as an adhesion mediator or molecular glue. A molecular assembly that is ultrastructurally very similar to AJ in epithelial cells, called asynaptic adherens junction, also provides mechanical stability at synapses. N-cadherin transmembrane proteins on the surface of either cell form clustered homophilic interactions in the synaptic cleft which are stabilised by their linkage to the microfilament system via a set of intracellular adapter proteins. An action potential arriving at the presynaptic membrane triggers the fusion of neurotransmitter-loaded vesicles with the axonal plasma membrane. The neurotransmitters are released into the synaptic cleft, diffuse very fast across this narrow extracellular space and bind to corresponding transmitter-gated ion channels on the postsynaptic membrane. Upon channel opening, the associated influx of ions depolarises the cell and thereby generates an action potential that can now migrate along the membrane of the postsynaptic nerve or muscle cell. Any excess of the neurotransmitter in the synaptic cleft that is no longer needed is quickly removed and the state of the synaptic junction is regenerated. Taken together, synaptic junctions provide the molecular requirements to transmit electrical impulses from one cell to the next via a burst of a transmitter molecule secreted into a defined volume close to the receiver membrane where the corresponding receptors are located.
Gap Junctions (GJ) and Plasmodesmata:
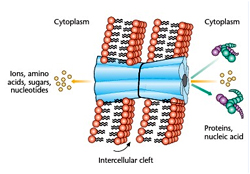
Accordingly, the cells may share small metabolites and ions but not macromolecules like proteins, deoxyribonucleic acid or ribonucleic acid. The term electrical synapses, in contrast to the chemical synapses described in the preceding paragraph, arose because GJ allow a free flow of ions from one cell to the next through the gap junction channels, which provides the requirements to transmit electrical signals without making use of chemical transmitters. This mode of action potential propagation is faster than the transmitter-based mechanism described above in neural synapses. Heart muscle cells are extensively interconnected by GJ, which provides the basis for highly synchronized contraction of the heart muscle. The participating cells can adjust the permeability of the gap junction channels. When the connexon hemichannels are open, the six connexin proteins are somewhat tilted with respect to the direction of the channel. A sudden increase of the intracellular Ca2+ concentration or a drop of pH provides conformational changes within the connexins, which eventually change their tilt angles and thereby close the channel. This is a rather important safety precaution because it avoids that breakdown of the osmotic balance in one cell, for instance when the plasma membrane has become permeable for any reason, propagates into all neighboring cells and destroys the tissue.
Plasmodesmata between plant cells have a very similar function, although the structure is rather different. This different structural solution for the same problem is apparently necessary because the rather thick cell wall does not allow plant cells to get into sufficiently intimate contact to build gap junction-like structures in which proteins bridge between the two cytoplasms. At plasmodesmata, the otherwise continuous cell wall around plant cells forms point-like openings at which the plasma membranes of neighbouring cells fuse. This provides a continuous channel lined by the plasma membrane, which is roughly 30–50 nm in diameter (compared to 1.5 nm for gap junction channels). To reduce the size of this connection and to provide some size selectivity, a membrane vesicle (desmotubule) that has budded off the endoplasmic reticulum acts like a cork in a bottle. This plugging of the intercellular connection slows down diffusional exchange and only allows sufficiently small molecules to migrate into neighbouring cells.
References
- Maeda S,Tsukihara T (2011) Structure of the Gap Junction Channel and its Implications for its Biological Functions. Cellular and Molecular Life Sciences 68(7); 1115-1129.
- Steed E, Balda MS and Matter K (2010) Dynamics and Functions of Tight Junctions. Trends in Cell Biology 20(3); 142-149.
- Ebnet K (2008) Organization of Multiprotein Complexes at Cell-cell Junctions. Histochemistry and Cell Biology 130; 1-20.
- Niessen CM and Gottardi CJ (2008) Molecular Components of the Adherens Junction. Biochemica et Biophysica Acta 1778; 562-571.
- Gumbiner BM (2005) Regulation of Cadherin-mediated Adhesion in Morphogenesis. Nature Review of Molecular Cell Biology 6; 622-634.
Published date : 14 May 2014 12:04PM