Cell Division
Sakshi Education
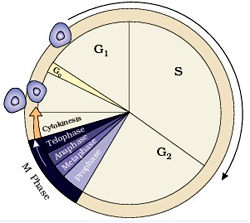
New cells are generated from parent cells by a process called cell division. All living cells have the capacity to divide into two identical daughter cells, by a process called binary fission (a mitotic-like process) (in prokaryotic cells) or mitosis (in eukaryotic cells).
Prokaryotic cells are simple in structure. They contain non-membranous organelles, lack a cell nucleus, and have a simplistic genome: only one circular chromosome of limited size. Therefore, prokaryotic cell division by a process known as binary fission, is fast. The chromosome is duplicated prior to division. The two copies of the chromosome attach to opposing sides of the cellular membrane. Cytokinesis, the physical separation of the cell, occurs immediately.
Many organisms reproduce by binary fissions, such as:
Mitosis is the process by which a cell separates its duplicated genome into two identical halves. It is generally followed immediately by cytokinesis which divides the cytoplasm and cell membrane. This results in two identical daughter cells with a roughly equal distribution of organelles and other cellular components. The word "mitosis" means "threads" and it refers to the threadlike appearance of chromosomes as the cell prepares to divide.
The time period in which cells replicate their chromosomal DNA and subsequently divide to give rise to two new cells is called Cell cycle. The period between cell divisions is called interphase which is far from the general convention of being an actual resting stage and in fact witnesses a heavy activity of synthesizing the ribonucleic acid (RNA) and proteins required for the subsequent stages of the cell cycle.
Mitosis and cytokinesis together is defined as the mitotic (M) phase of the cell cycle, the division of the mother cell into two daughter cells, each the genetic equivalent of the parent cell. The process of DNA replication occurs during ‘S’ (synthesis) phase of interphase and the content of DNA is doubled by G2 phase. Replication of chromosomes is actually replication of the DNA double helix which occurs at a ‘replication fork’, involving a host of different enzymes. After replication in the ‘S’ phase, there is a gap (‘G’) phase before the cell enters prophase, the first stage of mitosis. Mitosis consists of five morphologically distinct phases
Prophase
Prophase is the first stage in mitosis, occurring after the conclusion of the G2 portion of interphase. In the S and G2 phases the new DNA molecules formed are not distinct but interwined. During prophase, the parent cell chromosomes condense and become thousands of times more compact than they were during interphase. Thus initiation of condensation of chromosomal material marks the beginning of prophase. The chromosomal material becomes untangled during the process of chromatin condensation.
As each duplicated chromosome consists of two identical sister chromatids joined at a point called the centromere, these structures now appear as X-shaped bodies when viewed under a microscope. Several DNA binding proteins catalyze the condensation process, including cohesin and condensin. Cohesin forms rings that hold the sister chromatids together, whereas condensin forms rings that coil the chromosomes into highly compact forms. The centriole, which had undergone duplication during S phase of interphase, now begins to move towards opposite poles of the cell facilitating microtubules to assemble between them gradually, forming the network called mitotic spindle that will later pull the duplicated chromosomes apart.
The completion of prophase can thus be marked by the following characteristic events:
Condensation of chromatin to form compact mitotic chromosomes.
When prophase is complete, the cell enters prometaphase — the second stage of mitosis. During prometaphase, phosphorylation of nuclear lamins by M-CDK causes the nuclear membrane to break down into numerous small vesicles. As a result, the spindle microtubules now have direct access to the genetic material of the cell.
Each microtubule is highly dynamic, growing outward from the centrosome and collapsing backward as it tries to locate a chromosome. Eventually, the microtubules find their targets and connect to each chromosome at its kinetochore, a complex of proteins positioned at the centromere. The actual number of microtubules that attach to a kinetochore varies between species, but at least one microtubule from each pole attaches to the kinetochore of each chromosome. A tug-of-war then ensues as the chromosomes move back and forth toward the two poles.
At the end of prometaphase, cell organelles like golgi complexes, endoplasmic reticulum, nucleolus and the nuclear envelope appears to be dissolved when viewed under a microscope.
Metaphase
As prometaphase ends and metaphase begins, condensation of chromosomes is completed and they can be observed clearly under the microscope. Morphology of metaphase chromosome made up of two sister chromatids, which are held together by the centromere is most easily studied at this stage. Small disc-shaped structures present at the surface of the centromeres are called Kinetochores. Kinetochore microtubules attach the chromosomes to the spindle pole; interpolar microtubules extend from the spindle pole across the equator, almost to the opposite spindle pole; and astral microtubules extend from the spindle pole to the cell membrane. These structures move the chromosomes into position at the centre of the cell.
Hence, the metaphase is characterised by all the chromosomes coming to lie at the equator. Every chromosome has at least two microtubules extending from its kinetochore — with at least one microtubule connected to each pole. At this point, the tension within the cell becomes balanced, and the chromosomes no longer move back and forth. In addition, the spindle is now complete, and three groups of spindle microtubules are apparent.The plane of alignment of the chromosomes at metaphase is referred to as the metaphase plate. The key features of metaphase are:
Metaphase leads to anaphase, during which each chromosome arranged at the metaphase plate is split simultaneously and the two daughter chromatids, now referred to as chromosomes of the future daughter nuclei. They begin to move towards opposite poles of the cell. Enzymatic breakdown of cohesin — which linked the sister chromatids together during prophase causes this separation to occur. Upon separation, every chromatid becomes an independent chromosome. Meanwhile, changes in microtubule length provide the mechanism for chromosome movement.
More specifically, in the first part of anaphase — sometimes called anaphase A — the kinetochore microtubules shorten and draw the chromosomes toward the spindle poles. Then, in the second part of anaphase — sometimes called anaphase B — the astral microtubules that are anchored to the cell membrane pull the poles further apart and the interpolar microtubules slide past each other, exerting additional pull on the chromosomes. As each chromosome moves away from the equatorial plate, the centromere of each chromosome is towards the pole and hence at the leading edge, with the arms of the chromosome trailing behind. Thus, anaphase stage is characterised by the following key events:
Centromeres split and chromatids separate.
Chromatids move to opposite poles.
Telophase
During telophase, the chromosomes that arrive at the cell poles decondense and lose their individuality. Thereby the individual chromosomes can no longer be seen and chromatin material tends to collect in a mass in the two poles.
Further the mitotic spindle disassembles, and the vesicles that contain fragments of the original nuclear membrane assemble around the two sets of chromosomes. Phosphatases then dephosphorylate the lamins at each end of the cell. This dephosphorylation results in the formation of a new nuclear membrane around each group of chromosomes. This is the stage which shows the following key events:
Cytokinesis is the physical process that finally splits the parent cell into two identical daughter cells. In an animal cell, the cell membrane pinches in at the cell equator, forming a cleft called the cleavage furrow. The furrow gradually deepens and ultimately joins in the centre dividing the cell cytoplasm into two. The position of the furrow depends on the position of the astral and interpolar microtubules during anaphase. The cleavage furrow forms because of the action of a contractile ring of overlapping actin and myosin filaments.
As the actin and myosin filaments move past each other, the contractile ring becomes smaller, akin to pulling a drawstring at the top of a purse. When the ring reaches its smallest point, the cleavage furrow completely bisects the cell at its center, resulting in two separate daughter cells of equal size
Plant cells however, are enclosed by a relatively inextensible cell wall, therefore they undergo cytokinesis by a different mechanism. In plant cells, wall formation starts in the centre of the cell and grows outward to meet the existing lateral walls. The formation of the new cell wall begins with the formation of a simple precursor, called the cell-plate that represents the middle lamella between the walls of two adjacent cells. At the time of cytoplasmic division, organelles like mitochondria and plastids get distributed between the two daughter cells. In some organisms karyokinesis is not followed by cytokinesis as a result of which multinucleate condition arises leading to the formation of syncytium (e.g., liquid endosperm in coconut).
Significance of Mitosis
The production of offspring by sexual reproduction includes the fusion of two gametes, each with a complete haploid set of chromosomes. Gametes are formed from specialised diploid cells. This specialised kind of cell division that reduces the chromosome number by half results in the production of haploid daughter cells. This kind of division is called meiosis.
Meiosis, derived from the Greek word meioun, meaning "to make small," thus refers to the specialized process by which germ cells divide to produce gametes. Because the chromosome number of a species remains the same from one generation to the next, the chromosome number of germ cells must be reduced by half during meiosis. To accomplish this feat, meiosis, unlike mitosis, involves a single round of DNA replication followed by two rounds of cell division.
Organisms that reproduce sexually are thought to have an advantage over organisms that reproduce asexually, because novel combinations of genes are possible in each generation. Furthermore, with few exceptions, each individual in a population of sexually reproducing organisms has a distinct genetic composition. This is due to genetic recombination occurring during meiosis. Therefore Meiosis unlike mitosis involves the process of recombination, during which chromosomes exchange segments with one another. As a result, the gametes produced during meiosis are genetically unique.
Meiosis ensures the production of haploid phase in the life cycle of sexually reproducing organisms whereas fertilisation restores the diploid phase. We come across meiosis during gametogenesis in plants and animals. This leads to the formation of haploid gametes.
Meiosis involves two sequential cycles of nuclear and cell division called meiosis I and meiosis II but only a single cycle of DNA replication. Meiosis I is a unique cell division that occurs only in germ cells; meiosis II is similar to a mitotic division. Before germ cells enter meiosis, they are generally diploid, meaning that they have two homologous copies of each chromosome. Then, just before a germ cell enters meiosis, it duplicates its DNA at the S phase so that the cell contains four DNA copies distributed between two pairs of homologous chromosomes. Thus Meiosis I is initiated after the parental chromosomes have replicated to produce identical sister chromatids.
Meiotic events can be grouped under the following phases:
Meiosis I Meiosis II
Prophase I Prophase II
Metaphase I Metaphase II
Anaphase I Anaphase II
Telophase I Telophase II
Meiosis I
Compared to mitosis, which can take place in a matter of minutes, meiosis is a slow process, largely because of the time that the cell spends in prophase I. Prophase I is the longest and arguably most important segment of meiosis, because recombination occurs during this interval.
Prophase I
Prophase I has been divided into five phases, based upon the appearance of the meiotic chromosomes. First phase is described as leptotene (from the Greek for "thin threads") phase, which is followed sequentially by the zygotene (from the Greek for "paired threads"), pachytene (from the Greek for "thick threads"), and diplotene (from the Greek for "two threads") phases.
During leptotene stage the chromosomes become gradually visible under the light microscope. The compaction of chromosomes continues throughout leptotene.
This is followed by the second stage of prophase I called zygotene. During this stage chromosomes start pairing together and this process of association is called synapsis. Such paired chromosomes are called homologous chromosomes. Pairing of homologous chromosomes occurs with the appearance of double-stranded breaks (DSBs) in the DNA. The formation of DSBs is catalyzed by highly conserved proteins with topoisomerase. Following the DSBs, one DNA strand is trimmed back, leaving a 3'-overhang that "invades" a homologous sequence on another chromatid. As the invading strand is extended, a remarkable structure called synaptonemal complex (SC) develops around the paired homologues and holds them in close register, or synapsis. The complex formed by a pair of synapsed homologous chromosomes is called a bivalent or a tetrad.
However, these are more clearly visible at the next stage. The first two stages of prophase I are relatively short-lived compared to the next stage that is pachytene. During this stage bivalent chromosome now clearly appears as tetrads. The stability of the SC increases as the invading strand first extends into the homologue and then is recaptured by the broken chromatid, forming double Holliday junctions. Bridges approximately 400 nanometers long begin to form between the paired homologues following the DSB. Only a fraction of these bridges will mature into SC; moreover, not all Holliday junctions will mature into crossover sites. Crossing over is the exchange of genetic material between two homologous chromosomes. Crossing over is also an enzyme-mediated process and the enzyme involved is called recombinase. Crossing over leads to recombination of genetic material on the two chromosomes.
Recombination will thus occur at only a few sites along each chromosome, and the products of the crossover will become visible as chiasmata in diplotene after the SC has disappeared.
Recombination between homologous chromosomes is completed by the end of pachytene, leaving the chromosomes linked at the sites of crossing over.
The beginning of diplotene is recognised by the dissolution of the synaptonemal complex and the tendency of the recombined homologous chromosomes of the bivalents to separate from each other except at the sites of crossovers. These X-shaped structures, are called chiasmata. In oocytes of some vertebrates, diplotene can last for months or years.
The final stage of meiotic prophase I is diakinesis. This is marked by terminalisation of chiasmata. During this phase the chromosomes are fully condensed and the meiotic spindle is assembled to prepare the homologous chromosomes for separation. By the end of diakinesis, the nucleolus disappears and the nuclear envelope also breaks down. Diakinesis represents transition to metaphase.
Metaphase I
At the end of prometaphase I, meiotic cells enter metaphase I. Here, in sharp contrast to mitosis, pairs of homologous chromosomes line up opposite each other on the metaphase plate, with the kinetochores on sister chromatids facing the same pole. The microtubules from the opposite poles of the spindle attach to the pair of homologous chromosomes. Pairs of sex chromosomes also align on the metaphase plate. In human males, the Y chromosome pairs and crosses over with the X chromosome. These crossovers are possible because the X and Y chromosomes have small regions of similarity near their tips. Crossover between these homologous regions ensures that the sex chromosomes will segregate properly when the cell divides.
Anaphase I
The pairs of homologous chromosomes separate to different daughter cells while sister chromatids remain associated at their centromeres.
Before the pairs can separate, however, the crossovers between chromosomes must be resolved and meiosis-specific cohesins must be released from the arms of the sister chromatids. Failure to separate the pairs of chromosomes to different daughter cells is referred to as nondisjunction, and it is a major source of aneuploidy. Overall, aneuploidy appears to be a relatively frequent event in humans. In fact, the frequency of aneuploidy in humans has been estimated to be as high as 10% to 30%, and this frequency increases sharply with maternal age.
Telophase I
The nuclear membrane and nucleolus reappear, cytokinesis follows and this is called as diad of cells . Although in many cases the chromosomes do undergo some dispersion, they do not reach the extremely extended state of the interphase nucleus. The stage between the two meiotic divisions is called interkinesis and is generally short lived. Interkinesis is followed by prophase II, a much simpler prophase than prophase I.
Meiosis II
Following meiosis I, the daughter cells enter meiosis II without passing through interphase or replicating their DNA. Meiosis II resembles a mitotic division, except that the chromosome number has been reduced by half. Thus, the products of meiosis II are four haploid cells that contain a single copy of each chromosome.
Prophase II
Meiosis II is initiated immediately after cytokinesis, usually before the chromosomes have fully elongated. In contrast to meiosis I, meiosis II resembles a normal mitosis. The nuclear membrane disappears by the end of prophase II. The chromosomes again become compact.
Metaphase II
At this stage the chromosomes align at the equator and the microtubules from opposite poles of the spindle get attached to the kinetochores of sister chromatids.
Anaphase II
It begins with the simultaneous splitting of the centromere of each chromosome (which was holding the sister chromatids together), allowing them to move toward opposite poles of the cell (Figure 10.4).
Telophase II
Meiosis ends with telophase II, in which the two groups of chromosomes once again get enclosed by a nuclear envelope; cytokinesis follows resulting in the formation of tetrad of cells i.e., four haploid daughter cells.
SIGNIFICANCE OF MEIOSIS:
Prokaryotic cells are simple in structure. They contain non-membranous organelles, lack a cell nucleus, and have a simplistic genome: only one circular chromosome of limited size. Therefore, prokaryotic cell division by a process known as binary fission, is fast. The chromosome is duplicated prior to division. The two copies of the chromosome attach to opposing sides of the cellular membrane. Cytokinesis, the physical separation of the cell, occurs immediately.
Many organisms reproduce by binary fissions, such as:
- Bacteria (for example, Rickettsia species, that cause diseases such as Rocky Mountain spotted fever)
- Most protists (for example, Amoeba proteus)
- Entamoeba histolytica (a protozoan that is a human intestinal parasite)
- Pyrodictium abyssi (an anaerobic hyperthermophilic archaea of deep-sea hydrothermal vents)
- Schizosaccharomyces pombe (a fungal organism that is a species of yeast)
Mitosis is the process by which a cell separates its duplicated genome into two identical halves. It is generally followed immediately by cytokinesis which divides the cytoplasm and cell membrane. This results in two identical daughter cells with a roughly equal distribution of organelles and other cellular components. The word "mitosis" means "threads" and it refers to the threadlike appearance of chromosomes as the cell prepares to divide.
The time period in which cells replicate their chromosomal DNA and subsequently divide to give rise to two new cells is called Cell cycle. The period between cell divisions is called interphase which is far from the general convention of being an actual resting stage and in fact witnesses a heavy activity of synthesizing the ribonucleic acid (RNA) and proteins required for the subsequent stages of the cell cycle.
Mitosis and cytokinesis together is defined as the mitotic (M) phase of the cell cycle, the division of the mother cell into two daughter cells, each the genetic equivalent of the parent cell. The process of DNA replication occurs during ‘S’ (synthesis) phase of interphase and the content of DNA is doubled by G2 phase. Replication of chromosomes is actually replication of the DNA double helix which occurs at a ‘replication fork’, involving a host of different enzymes. After replication in the ‘S’ phase, there is a gap (‘G’) phase before the cell enters prophase, the first stage of mitosis. Mitosis consists of five morphologically distinct phases
- Prophase
- Prometaphase
- Metaphase
- Anaphase
- Telophase
Prophase
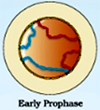
As each duplicated chromosome consists of two identical sister chromatids joined at a point called the centromere, these structures now appear as X-shaped bodies when viewed under a microscope. Several DNA binding proteins catalyze the condensation process, including cohesin and condensin. Cohesin forms rings that hold the sister chromatids together, whereas condensin forms rings that coil the chromosomes into highly compact forms. The centriole, which had undergone duplication during S phase of interphase, now begins to move towards opposite poles of the cell facilitating microtubules to assemble between them gradually, forming the network called mitotic spindle that will later pull the duplicated chromosomes apart.
The completion of prophase can thus be marked by the following characteristic events:
Condensation of chromatin to form compact mitotic chromosomes.
- Chromosomes are seen to be composed of two chromatids attached together at the centromere.
- Initiation of assembly of the mitotic spindle with the aid of cytoskeletal elements like microtubules.
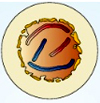
Each microtubule is highly dynamic, growing outward from the centrosome and collapsing backward as it tries to locate a chromosome. Eventually, the microtubules find their targets and connect to each chromosome at its kinetochore, a complex of proteins positioned at the centromere. The actual number of microtubules that attach to a kinetochore varies between species, but at least one microtubule from each pole attaches to the kinetochore of each chromosome. A tug-of-war then ensues as the chromosomes move back and forth toward the two poles.
At the end of prometaphase, cell organelles like golgi complexes, endoplasmic reticulum, nucleolus and the nuclear envelope appears to be dissolved when viewed under a microscope.
Metaphase
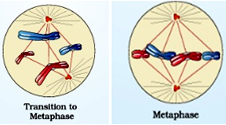
Hence, the metaphase is characterised by all the chromosomes coming to lie at the equator. Every chromosome has at least two microtubules extending from its kinetochore — with at least one microtubule connected to each pole. At this point, the tension within the cell becomes balanced, and the chromosomes no longer move back and forth. In addition, the spindle is now complete, and three groups of spindle microtubules are apparent.The plane of alignment of the chromosomes at metaphase is referred to as the metaphase plate. The key features of metaphase are:
- Spindle fibres attach to kinetochores of chromosomes.
- Chromosomes are moved to spindle equator and get aligned along metaphase plate through spindle fibres to both poles.
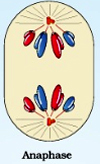
More specifically, in the first part of anaphase — sometimes called anaphase A — the kinetochore microtubules shorten and draw the chromosomes toward the spindle poles. Then, in the second part of anaphase — sometimes called anaphase B — the astral microtubules that are anchored to the cell membrane pull the poles further apart and the interpolar microtubules slide past each other, exerting additional pull on the chromosomes. As each chromosome moves away from the equatorial plate, the centromere of each chromosome is towards the pole and hence at the leading edge, with the arms of the chromosome trailing behind. Thus, anaphase stage is characterised by the following key events:
Centromeres split and chromatids separate.
Chromatids move to opposite poles.
Telophase
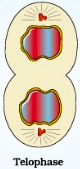
Further the mitotic spindle disassembles, and the vesicles that contain fragments of the original nuclear membrane assemble around the two sets of chromosomes. Phosphatases then dephosphorylate the lamins at each end of the cell. This dephosphorylation results in the formation of a new nuclear membrane around each group of chromosomes. This is the stage which shows the following key events:
- Chromosomes cluster at opposite spindle poles and their identity is lost as discrete elements.
- Nuclear envelope assembles around the chromosome clusters.
- Nucleolus, golgi complex and ER reform.
Cytokinesis is the physical process that finally splits the parent cell into two identical daughter cells. In an animal cell, the cell membrane pinches in at the cell equator, forming a cleft called the cleavage furrow. The furrow gradually deepens and ultimately joins in the centre dividing the cell cytoplasm into two. The position of the furrow depends on the position of the astral and interpolar microtubules during anaphase. The cleavage furrow forms because of the action of a contractile ring of overlapping actin and myosin filaments.
As the actin and myosin filaments move past each other, the contractile ring becomes smaller, akin to pulling a drawstring at the top of a purse. When the ring reaches its smallest point, the cleavage furrow completely bisects the cell at its center, resulting in two separate daughter cells of equal size
Plant cells however, are enclosed by a relatively inextensible cell wall, therefore they undergo cytokinesis by a different mechanism. In plant cells, wall formation starts in the centre of the cell and grows outward to meet the existing lateral walls. The formation of the new cell wall begins with the formation of a simple precursor, called the cell-plate that represents the middle lamella between the walls of two adjacent cells. At the time of cytoplasmic division, organelles like mitochondria and plastids get distributed between the two daughter cells. In some organisms karyokinesis is not followed by cytokinesis as a result of which multinucleate condition arises leading to the formation of syncytium (e.g., liquid endosperm in coconut).
Significance of Mitosis
- Mitosis or the equational division is usually restricted to the diploid cells only. However, in some lower plants and in some social insects haploid cells also divide by mitosis.
- Duplication of somatic cells by mitotic division is a fundamental cellular function that provides a contimous supply of cells to create the body of an otganism during its life cycle. Thus the growth of multicellular organisms is due to mitosis. Cell growth results in disturbing the ratio between the nucleus and the cytoplasm. It therefore becomes essential for the cell to divide to restore the nucleo-cytoplasmic ratio. A very significant contribution of mitosis is cell repair. The cells of the upper layer of the epidermis, cells of the lining of the gut, and blood cells are being constantly replaced. Mitotic divisions in the meristematic tissues – the apical and the lateral cambium, result in a continuous growth of plants throughout their life.
The production of offspring by sexual reproduction includes the fusion of two gametes, each with a complete haploid set of chromosomes. Gametes are formed from specialised diploid cells. This specialised kind of cell division that reduces the chromosome number by half results in the production of haploid daughter cells. This kind of division is called meiosis.
Meiosis, derived from the Greek word meioun, meaning "to make small," thus refers to the specialized process by which germ cells divide to produce gametes. Because the chromosome number of a species remains the same from one generation to the next, the chromosome number of germ cells must be reduced by half during meiosis. To accomplish this feat, meiosis, unlike mitosis, involves a single round of DNA replication followed by two rounds of cell division.
Organisms that reproduce sexually are thought to have an advantage over organisms that reproduce asexually, because novel combinations of genes are possible in each generation. Furthermore, with few exceptions, each individual in a population of sexually reproducing organisms has a distinct genetic composition. This is due to genetic recombination occurring during meiosis. Therefore Meiosis unlike mitosis involves the process of recombination, during which chromosomes exchange segments with one another. As a result, the gametes produced during meiosis are genetically unique.
Meiosis ensures the production of haploid phase in the life cycle of sexually reproducing organisms whereas fertilisation restores the diploid phase. We come across meiosis during gametogenesis in plants and animals. This leads to the formation of haploid gametes.
Meiosis involves two sequential cycles of nuclear and cell division called meiosis I and meiosis II but only a single cycle of DNA replication. Meiosis I is a unique cell division that occurs only in germ cells; meiosis II is similar to a mitotic division. Before germ cells enter meiosis, they are generally diploid, meaning that they have two homologous copies of each chromosome. Then, just before a germ cell enters meiosis, it duplicates its DNA at the S phase so that the cell contains four DNA copies distributed between two pairs of homologous chromosomes. Thus Meiosis I is initiated after the parental chromosomes have replicated to produce identical sister chromatids.
Meiotic events can be grouped under the following phases:
Meiosis I Meiosis II
Prophase I Prophase II
Metaphase I Metaphase II
Anaphase I Anaphase II
Telophase I Telophase II
Meiosis I
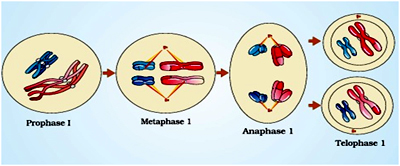
Prophase I
Prophase I has been divided into five phases, based upon the appearance of the meiotic chromosomes. First phase is described as leptotene (from the Greek for "thin threads") phase, which is followed sequentially by the zygotene (from the Greek for "paired threads"), pachytene (from the Greek for "thick threads"), and diplotene (from the Greek for "two threads") phases.
During leptotene stage the chromosomes become gradually visible under the light microscope. The compaction of chromosomes continues throughout leptotene.
This is followed by the second stage of prophase I called zygotene. During this stage chromosomes start pairing together and this process of association is called synapsis. Such paired chromosomes are called homologous chromosomes. Pairing of homologous chromosomes occurs with the appearance of double-stranded breaks (DSBs) in the DNA. The formation of DSBs is catalyzed by highly conserved proteins with topoisomerase. Following the DSBs, one DNA strand is trimmed back, leaving a 3'-overhang that "invades" a homologous sequence on another chromatid. As the invading strand is extended, a remarkable structure called synaptonemal complex (SC) develops around the paired homologues and holds them in close register, or synapsis. The complex formed by a pair of synapsed homologous chromosomes is called a bivalent or a tetrad.
However, these are more clearly visible at the next stage. The first two stages of prophase I are relatively short-lived compared to the next stage that is pachytene. During this stage bivalent chromosome now clearly appears as tetrads. The stability of the SC increases as the invading strand first extends into the homologue and then is recaptured by the broken chromatid, forming double Holliday junctions. Bridges approximately 400 nanometers long begin to form between the paired homologues following the DSB. Only a fraction of these bridges will mature into SC; moreover, not all Holliday junctions will mature into crossover sites. Crossing over is the exchange of genetic material between two homologous chromosomes. Crossing over is also an enzyme-mediated process and the enzyme involved is called recombinase. Crossing over leads to recombination of genetic material on the two chromosomes.
Recombination will thus occur at only a few sites along each chromosome, and the products of the crossover will become visible as chiasmata in diplotene after the SC has disappeared.
Recombination between homologous chromosomes is completed by the end of pachytene, leaving the chromosomes linked at the sites of crossing over.
The beginning of diplotene is recognised by the dissolution of the synaptonemal complex and the tendency of the recombined homologous chromosomes of the bivalents to separate from each other except at the sites of crossovers. These X-shaped structures, are called chiasmata. In oocytes of some vertebrates, diplotene can last for months or years.
The final stage of meiotic prophase I is diakinesis. This is marked by terminalisation of chiasmata. During this phase the chromosomes are fully condensed and the meiotic spindle is assembled to prepare the homologous chromosomes for separation. By the end of diakinesis, the nucleolus disappears and the nuclear envelope also breaks down. Diakinesis represents transition to metaphase.
Metaphase I
At the end of prometaphase I, meiotic cells enter metaphase I. Here, in sharp contrast to mitosis, pairs of homologous chromosomes line up opposite each other on the metaphase plate, with the kinetochores on sister chromatids facing the same pole. The microtubules from the opposite poles of the spindle attach to the pair of homologous chromosomes. Pairs of sex chromosomes also align on the metaphase plate. In human males, the Y chromosome pairs and crosses over with the X chromosome. These crossovers are possible because the X and Y chromosomes have small regions of similarity near their tips. Crossover between these homologous regions ensures that the sex chromosomes will segregate properly when the cell divides.
Anaphase I
The pairs of homologous chromosomes separate to different daughter cells while sister chromatids remain associated at their centromeres.
Before the pairs can separate, however, the crossovers between chromosomes must be resolved and meiosis-specific cohesins must be released from the arms of the sister chromatids. Failure to separate the pairs of chromosomes to different daughter cells is referred to as nondisjunction, and it is a major source of aneuploidy. Overall, aneuploidy appears to be a relatively frequent event in humans. In fact, the frequency of aneuploidy in humans has been estimated to be as high as 10% to 30%, and this frequency increases sharply with maternal age.
Telophase I
The nuclear membrane and nucleolus reappear, cytokinesis follows and this is called as diad of cells . Although in many cases the chromosomes do undergo some dispersion, they do not reach the extremely extended state of the interphase nucleus. The stage between the two meiotic divisions is called interkinesis and is generally short lived. Interkinesis is followed by prophase II, a much simpler prophase than prophase I.
Meiosis II
Following meiosis I, the daughter cells enter meiosis II without passing through interphase or replicating their DNA. Meiosis II resembles a mitotic division, except that the chromosome number has been reduced by half. Thus, the products of meiosis II are four haploid cells that contain a single copy of each chromosome.
Prophase II
Meiosis II is initiated immediately after cytokinesis, usually before the chromosomes have fully elongated. In contrast to meiosis I, meiosis II resembles a normal mitosis. The nuclear membrane disappears by the end of prophase II. The chromosomes again become compact.
Metaphase II
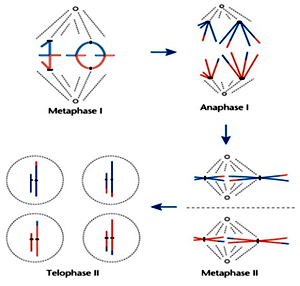
Anaphase II
It begins with the simultaneous splitting of the centromere of each chromosome (which was holding the sister chromatids together), allowing them to move toward opposite poles of the cell (Figure 10.4).
Telophase II
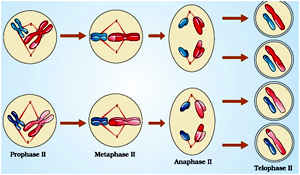
SIGNIFICANCE OF MEIOSIS:
- Meiosis is the mechanism by which conservation of specific chromosome number of each species is achieved across generations in sexually reproducing organisms.
- As meiosis involves crossing over leading to recombination, to generate variation. Variations act as an important source of evolution.
- The products of meiosis II are four haploid cells that contain a single copy of each chromosome. However in mammals, the number of viable gametes obtained from meiosis differs between males and females. In males, four haploid spermatids of similar size are produced from each spermatogonium. In females, however, the cytoplasmic divisions that occur during meiosis are very asymmetric. Fully grown oocytes within the ovary are already much larger than sperm, and the future egg retains most of this volume as it passes through meiosis. As a consequence, only one functional oocyte is obtained from each female meiosis. The other three haploid cells are pinched off from the oocyte as polar bodies that contain very little cytoplasm.
- Lopez-Maury, L., Marguerat, S., & Bahler, J. (2008) Tuning Gene Expression to Changing Environments: From Rapid Responses to Evolutionary Adaptation. Nature Reviews Genetics 9, 583–593.
- Gerton, J. L., & Hawley, R. S. (2005) Homologous Chromosome Interactions in Meiosis: Diversity amidst Conservation. Nature Reviews Genetics 6, 477–487
- Marston, A. L., & Amon, A. (2004) Meiosis: Cell-Cycle Controls Shuffle and Deal. Nature Reviews Molecular Cell Biology 5, 993–1008.
- Page, S. L., & Hawley, R. S. (2003) Chromosome Choreography: The Meiotic Ballet. Science 301, 785–789.
- Criqui, M.C & Genshik,P.(2002) Mitosis in Plants. Mitosis in Plants: How Far We Have Come at the Molecular Level? Curr.Opin. Plant Biol. 5, 487-493.
- Hassold, T., & Hunt, P. (2001) To Err (Meiotically) is Human: The Genesis of Human Aneuploidy. Nature Reviews Genetics 2, 280–291.
- Petes, T. D. (2001) Meiotic Recombination Hot Spots and Cold Spots. Nature Reviews Genetics 2, 360–369.
- Zickler, D., & Kleckner, N. (1999) Meiotic Chromosomes: Integrating Structure and Function. Annual Review of Genetics 33, 603–754.
Published date : 14 May 2014 03:03PM