Medium Access Sub Layer
Sakshi Education
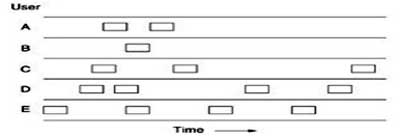
Random Access:
ALOHA:
ALOHA was developed by Norman Abramson and his colleagues at the University of Hawaii devised a solution for the channel allocation problem. Initially Abramson's work called the ALOHA system used ground-based radio broadcasting; the basic idea is applicable to any system in which uncoordinated users are competing for the use of a single shared channel. There are two versions of ALOHA: pure and slotted. They differ with respect to whether time is divided into discrete slots into which all frames must fit. Pure ALOHA does not require global time synchronization; slotted ALOHA does.
Pure ALOHA:
The basic idea of an ALOHA system is simple: let users transmit whenever they have data to be sent. There will be collisions, of course, and the colliding frames will be damaged. However, due to the feedback property of broadcasting, a sender can always find out whether its frame was destroyed by listening to the channel, the same way other users do. With a LAN, the feedback is immediate; with a satellite, there is a delay of 270 msec before the sender knows if the transmission was successful. If listening while transmitting is not possible for some reason, acknowledgements are needed. If the frame was destroyed, the sender just waits a random amount of time and sends it again. The waiting time must be random or the same frames will collide over and over, in lockstep. Systems in which multiple users share a common channel in a way that can lead to conflicts are widely known as contention systems.
A sketch of frame generation in an ALOHA system is given in Fig.1. We have made the frames all the same length because the throughput of ALOHA systems is maximized by having a uniform frame size rather than by allowing variable length frames.
Fig.1 In pure ALOHA, frames are transmitted at completely arbitrary times.
Whenever two frames try to occupy the channel at the same time, there will be a collision and both will be garbled. If the first bit of a new frame overlaps with just the last bit of a frame almost finished, both frames will be totally destroyed and both will have to be retransmitted later. The checksum cannot (and should not) distinguish between a total loss and a near miss.
Let the ''frame time'' denote the amount of time needed to transmit the standard, fixed length frame (i.e., the frame length divided by the bit rate). At this point, we assume that the infinite population of users generates new frames according to a Poisson distribution with mean N frames per frame time. (The infinite-population assumption is needed to ensure that N does no decrease as users become blocked.) If N > 1, the user community is generating frames at a higher rate than the channel can handle, and nearly every frame will suffer a collision. For reasonable throughput we would expect 0 < N < 1. In addition to the new frames, the stations also generate retransmissions of frames that previously suffered collisions. Let us further assume that the probability of k transmission attempts per frame time, old and new combined, is also Poisson, with mean G per frame time. Clearly, G=N. At low load (i.e., N 0), there will be few collisions, hence few retransmissions, so G N. At high load there will be many collisions, so G > N. Under all loads, the throughput, S, is just the offered load, G, times the probability, P0, of a transmission succeeding—that is, S = GP0, where P0 is the probability that a frame does not suffer a collision.
A frame will not suffer a collision if no other frames are sent within one frame time of its start, as shown in Fig.2.
Fig.2. Vulnerable period for the shaded frame
Under what conditions will the shaded frame arrive undamaged?
Let t be the time required to send a frame. If any other user has generated a frame between time t0 and t0+tr, the end of that frame will collide with the beginning of the shaded one. In fact, the shaded frame's fate was already sealed even before the first bit was sent, but since in pure ALOHA a station does not listen to the channel before transmitting, it has no way of knowing that another frame was already underway. Similarly, any other frame started between t0+t and t0+2t will bump into the end of the shaded frame. The probability that k frames are generated during a given frame time is given by the Poisson distribution:
Equation
So the probability of zero frames is just e-G. In an interval two frame times long, the mean number of frames generated is 2G. The probability of no other traffic being initiated during the entire vulnerable period is thus given by P0 = e -2G. Using S = GP0, we get
The relation between the offered traffic and the throughput is shown in Fig. 4-3. The maximum throughput occurs at G = 0.4, with S = 1/2e, which is about 0.184. In other words, the best we can hope for is a channel utilization of 18 per cent. This result is not very encouraging, but with everyone transmitting at will, we could hardly have expected a 100 per cent success rate.
Slotted ALOHA:
In 1972, Roberts published a method for doubling the capacity of an ALOHA system (Robert, 1972). His proposal was to divide time into discrete intervals, each interval corresponding to one frame. This approach requires the users to agree on slot boundaries. One way to achieve synchronization would be to have one special station emit a pip at the start of each interval, like a clock.
In Roberts' method, which has come to be known as slotted ALOHA, in contrast to Abramson's pure ALOHA, a computer is not permitted to send whenever a carriage return is typed. Instead, it is required to wait for the beginning of the next slot. Thus, the continuous pure ALOHA is turned into a discrete one. Since the vulnerable period is now halved, the probability of no other traffic during the same slot as our test frame is e-G which leads to equation
As you can see from Fig.3, slotted ALOHA peaks at G = 1, with a throughput of S=1/e or about
0.368, twice that of pure ALOHA. If the system is operating at G = 1, the probability of an empty slot is 0.368. The best we can hope for using slotted ALOHA is 37 percent of the slots empty, 37 percent successes, and 26 percent collisions. Operating at higher values of G reduces the number of empties but increases the number of collisions exponentially.
To see how this rapid growth of collisions with G comes about, consider the transmission of a test frame. The probability that it will avoid a collision is e-G, the probability that all the other users are silent in that slot. The probability of a collision is then just 1 – e-G. The probability of a transmission requiring exactly k attempts, (i.e., k – 1 collisions followed by one success) is
Fig.3 Throughput versus offered traffic for ALOHA systems.
The expected number of transmissions, E, per carriage return typed is then
As a result of the exponential dependence of E upon G, small increases in the channel load can drastically reduce its performance.
4.1.2 CSMA (Carrier Sense Multiple Access Protocols):
With slotted ALOHA, the best channel utilization that can be achieved is 1/e. This is hardly surprising, since with stations transmitting at will, without paying attention to what the other stations are doing, there are bound to be many collisions. In local area networks, however, it is possible for stations to detect what other stations are doing, and adapt their behavior accordingly. These networks can achieve a much better utilization than 1/e. In this section we will discuss some protocols for improving performance. Protocols in which stations listen for a carrier (i.e., a transmission) and act accordingly are called carrier sense protocols. A number of them have been proposed. Klein Rock and Tobagi (1974) have analyzed several such protocols in detail. Below we will mention several versions of the carrier sense protocols.
1.1-persistent CSMA:
The first carrier sense protocol that we will study here is called 1-persistent CSMA (Carrier Sense Multiple Access). When a station has data to send, it first listens to the channel to see if anyone else is transmitting at that moment. If the channel is busy, the station waits until it becomes idle. When the station detects an idle channel, it transmits a frame. If a collision occurs, the station waits a random amount of time and starts all over again. The protocol is called 1- persistent because the station transmits with a probability of 1 when it finds the channel idle.
The propagation delay has an important effect on the performance of the protocol. There is a small chance that just after a station begins sending, another station will become ready to send and sense the channel. If the first station's signal has not yet reached the second one, the latter will sense an idle channel and will also begin sending, resulting in a collision. The longer the propagation delay, the more important this effect becomes, and the worse the performance of the protocol. Even if the propagation delay is zero, there will still be collisions. If two stations become ready in the middle of a third station's transmission, both will wait politely until the transmission ends and then both will begin transmitting exactly simultaneously, resulting in a collision. If they were not so impatient, there would be fewer collisions. Even so, this protocol is far better than pure ALOHA because both stations have the decency to desist from interfering with the third station's frame. Intuitively, this approach will lead to a higher performance than pure ALOHA. Exactly the same holds for slotted ALOHA.
2. Non-persistent CSMA:
A second carrier sense protocol is non-persistent CSMA. In this protocol, a conscious attempt is made to be less greedy than in the previous one. Before sending, a station senses the channel. If no one else is sending, the station begins doing so itself. However, if the channel is already in use, the station does not continually sense it for the purpose of seizing it immediately upon detecting the end of the previous transmission. Instead, it waits a random period of time and then repeats the algorithm. Consequently, this algorithm leads to better channel utilization but longer delays than 1-persistent CSMA.
3. P-persistent CSMA:
The last protocol is p-persistent CSMA. It applies to slotted channels and works as follows. When a station becomes ready to send, it senses the channel. If it is idle, it transmits with a probability p. With a probability q = 1 - p, it defers until the next slot. If that slot is also idle, It either transmits or defers again, with probabilities p and q. This process is repeated until either the frame has been transmitted or another station has begun transmitting. In the latter case, the unlucky station acts as if there had been a collision (i.e., it waits a random time and starts again). If the station initially senses the channel busy, it waits until the next slot and applies the above algorithm. Figure 4 shows the computed throughput versus offered traffic for all three protocols, as well as for pure and slotted ALOHA.
Fig.4 Comparison of the channel utilization versus load for various random access protocols
4.1.3 CSMA with Collision Detection:
Persistent and nonpersistent CSMA protocols are clearly an improvement over ALOHA because they ensure that no station begins to transmit when it senses the channel busy. Another improvement is for stations to abort their transmissions as soon as they detect a collision. In other words, if two stations sense the channel to be idle and begin transmitting simultaneously, they will both detect the collision almost immediately. Rather than finish transmitting their frames, which are irretrievably garbled anyway, they should abruptly stop transmitting as soon as the collision is detected. Quickly terminating damaged frames saves time and bandwidth.
This protocol, known as CSMA/CD (CSMA with Collision Detection) is widely used on LANs in the MAC sub layer. In particular, it is the basis of the popular Ethernet LAN, so it is worth devoting some time to looking at it in detail. CSMA/CD, as well as many other LAN protocols, uses the conceptual model of Fig.4. At the point marked t0, a station has finished transmitting its frame. Any other station having a frame to send may now attempt to do so. If two or more stations decide to transmit simultaneously, there will be a collision. Collisions can be detected by looking at the power or pulse width of the received signal and comparing it to the transmitted signal.
Fig.4. CSMA/CD can be in one of three states: contention, transmission, oridle
After a station detects a collision, it aborts its transmission, waits a random period of time, and then tries again, assuming that no other station has started transmitting in the meantime. Therefore, our model for CSMA/CD will consist of alternating contention and transmission periods, with idle periods occurring when all stations are quiet (e.g., for lack of work).
Now let us look closely at the details of the contention algorithm. Suppose that two stations both begin transmitting at exactly time t0. How long will it take them to realize that there has been a collision? The answer to this question is vital to determining the length of the contention period and hence what the delay and throughput will be. The minimum time to detect the collision is then just the time it takes the signal to propagate from one station to the other.
Based on this reasoning, you might think that a station not hearing a collision for a time equal to the full cable propagation time after starting its transmission could be sure it had seized the cable. By ''seized,'' we mean that all other stations knew it was transmitting and would not interfere. This conclusion is wrong. Consider the following worst-case scenario. Let the time for a signal to propagate between the two farthest stations be. At t0, one station begins transmitting. At an instant before the signal arrives at the most distant station, that station also begins transmitting. Of course, it detects the collision almost instantly and stops, but the little noise burst caused by the collision does not get back to the original station until time. In other words, in the worst case a station cannot be sure that it has seized the channel until it has transmitted for without hearing a collision. For this reason we will model the contention interval as a slotted ALOHA system with slot width.
ALOHA:
ALOHA was developed by Norman Abramson and his colleagues at the University of Hawaii devised a solution for the channel allocation problem. Initially Abramson's work called the ALOHA system used ground-based radio broadcasting; the basic idea is applicable to any system in which uncoordinated users are competing for the use of a single shared channel. There are two versions of ALOHA: pure and slotted. They differ with respect to whether time is divided into discrete slots into which all frames must fit. Pure ALOHA does not require global time synchronization; slotted ALOHA does.
Pure ALOHA:
The basic idea of an ALOHA system is simple: let users transmit whenever they have data to be sent. There will be collisions, of course, and the colliding frames will be damaged. However, due to the feedback property of broadcasting, a sender can always find out whether its frame was destroyed by listening to the channel, the same way other users do. With a LAN, the feedback is immediate; with a satellite, there is a delay of 270 msec before the sender knows if the transmission was successful. If listening while transmitting is not possible for some reason, acknowledgements are needed. If the frame was destroyed, the sender just waits a random amount of time and sends it again. The waiting time must be random or the same frames will collide over and over, in lockstep. Systems in which multiple users share a common channel in a way that can lead to conflicts are widely known as contention systems.
A sketch of frame generation in an ALOHA system is given in Fig.1. We have made the frames all the same length because the throughput of ALOHA systems is maximized by having a uniform frame size rather than by allowing variable length frames.
Fig.1 In pure ALOHA, frames are transmitted at completely arbitrary times.
Whenever two frames try to occupy the channel at the same time, there will be a collision and both will be garbled. If the first bit of a new frame overlaps with just the last bit of a frame almost finished, both frames will be totally destroyed and both will have to be retransmitted later. The checksum cannot (and should not) distinguish between a total loss and a near miss.
Let the ''frame time'' denote the amount of time needed to transmit the standard, fixed length frame (i.e., the frame length divided by the bit rate). At this point, we assume that the infinite population of users generates new frames according to a Poisson distribution with mean N frames per frame time. (The infinite-population assumption is needed to ensure that N does no decrease as users become blocked.) If N > 1, the user community is generating frames at a higher rate than the channel can handle, and nearly every frame will suffer a collision. For reasonable throughput we would expect 0 < N < 1. In addition to the new frames, the stations also generate retransmissions of frames that previously suffered collisions. Let us further assume that the probability of k transmission attempts per frame time, old and new combined, is also Poisson, with mean G per frame time. Clearly, G=N. At low load (i.e., N 0), there will be few collisions, hence few retransmissions, so G N. At high load there will be many collisions, so G > N. Under all loads, the throughput, S, is just the offered load, G, times the probability, P0, of a transmission succeeding—that is, S = GP0, where P0 is the probability that a frame does not suffer a collision.
A frame will not suffer a collision if no other frames are sent within one frame time of its start, as shown in Fig.2.
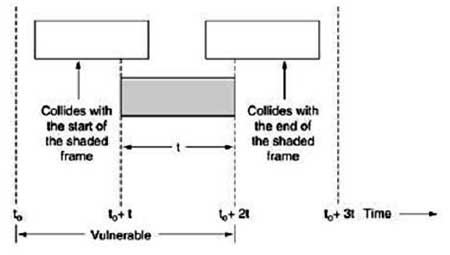
Fig.2. Vulnerable period for the shaded frame
Under what conditions will the shaded frame arrive undamaged?
Let t be the time required to send a frame. If any other user has generated a frame between time t0 and t0+tr, the end of that frame will collide with the beginning of the shaded one. In fact, the shaded frame's fate was already sealed even before the first bit was sent, but since in pure ALOHA a station does not listen to the channel before transmitting, it has no way of knowing that another frame was already underway. Similarly, any other frame started between t0+t and t0+2t will bump into the end of the shaded frame. The probability that k frames are generated during a given frame time is given by the Poisson distribution:
Equation
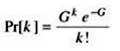
So the probability of zero frames is just e-G. In an interval two frame times long, the mean number of frames generated is 2G. The probability of no other traffic being initiated during the entire vulnerable period is thus given by P0 = e -2G. Using S = GP0, we get
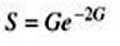
The relation between the offered traffic and the throughput is shown in Fig. 4-3. The maximum throughput occurs at G = 0.4, with S = 1/2e, which is about 0.184. In other words, the best we can hope for is a channel utilization of 18 per cent. This result is not very encouraging, but with everyone transmitting at will, we could hardly have expected a 100 per cent success rate.
Slotted ALOHA:
In 1972, Roberts published a method for doubling the capacity of an ALOHA system (Robert, 1972). His proposal was to divide time into discrete intervals, each interval corresponding to one frame. This approach requires the users to agree on slot boundaries. One way to achieve synchronization would be to have one special station emit a pip at the start of each interval, like a clock.
In Roberts' method, which has come to be known as slotted ALOHA, in contrast to Abramson's pure ALOHA, a computer is not permitted to send whenever a carriage return is typed. Instead, it is required to wait for the beginning of the next slot. Thus, the continuous pure ALOHA is turned into a discrete one. Since the vulnerable period is now halved, the probability of no other traffic during the same slot as our test frame is e-G which leads to equation
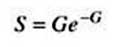
As you can see from Fig.3, slotted ALOHA peaks at G = 1, with a throughput of S=1/e or about
0.368, twice that of pure ALOHA. If the system is operating at G = 1, the probability of an empty slot is 0.368. The best we can hope for using slotted ALOHA is 37 percent of the slots empty, 37 percent successes, and 26 percent collisions. Operating at higher values of G reduces the number of empties but increases the number of collisions exponentially.
To see how this rapid growth of collisions with G comes about, consider the transmission of a test frame. The probability that it will avoid a collision is e-G, the probability that all the other users are silent in that slot. The probability of a collision is then just 1 – e-G. The probability of a transmission requiring exactly k attempts, (i.e., k – 1 collisions followed by one success) is
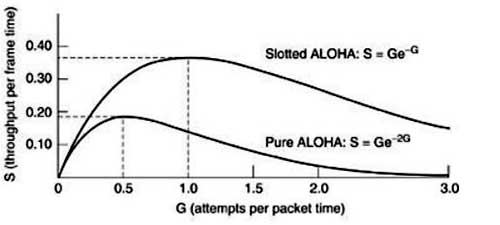
Fig.3 Throughput versus offered traffic for ALOHA systems.
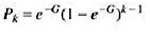
The expected number of transmissions, E, per carriage return typed is then

As a result of the exponential dependence of E upon G, small increases in the channel load can drastically reduce its performance.
4.1.2 CSMA (Carrier Sense Multiple Access Protocols):
With slotted ALOHA, the best channel utilization that can be achieved is 1/e. This is hardly surprising, since with stations transmitting at will, without paying attention to what the other stations are doing, there are bound to be many collisions. In local area networks, however, it is possible for stations to detect what other stations are doing, and adapt their behavior accordingly. These networks can achieve a much better utilization than 1/e. In this section we will discuss some protocols for improving performance. Protocols in which stations listen for a carrier (i.e., a transmission) and act accordingly are called carrier sense protocols. A number of them have been proposed. Klein Rock and Tobagi (1974) have analyzed several such protocols in detail. Below we will mention several versions of the carrier sense protocols.
1.1-persistent CSMA:
The first carrier sense protocol that we will study here is called 1-persistent CSMA (Carrier Sense Multiple Access). When a station has data to send, it first listens to the channel to see if anyone else is transmitting at that moment. If the channel is busy, the station waits until it becomes idle. When the station detects an idle channel, it transmits a frame. If a collision occurs, the station waits a random amount of time and starts all over again. The protocol is called 1- persistent because the station transmits with a probability of 1 when it finds the channel idle.
The propagation delay has an important effect on the performance of the protocol. There is a small chance that just after a station begins sending, another station will become ready to send and sense the channel. If the first station's signal has not yet reached the second one, the latter will sense an idle channel and will also begin sending, resulting in a collision. The longer the propagation delay, the more important this effect becomes, and the worse the performance of the protocol. Even if the propagation delay is zero, there will still be collisions. If two stations become ready in the middle of a third station's transmission, both will wait politely until the transmission ends and then both will begin transmitting exactly simultaneously, resulting in a collision. If they were not so impatient, there would be fewer collisions. Even so, this protocol is far better than pure ALOHA because both stations have the decency to desist from interfering with the third station's frame. Intuitively, this approach will lead to a higher performance than pure ALOHA. Exactly the same holds for slotted ALOHA.
2. Non-persistent CSMA:
A second carrier sense protocol is non-persistent CSMA. In this protocol, a conscious attempt is made to be less greedy than in the previous one. Before sending, a station senses the channel. If no one else is sending, the station begins doing so itself. However, if the channel is already in use, the station does not continually sense it for the purpose of seizing it immediately upon detecting the end of the previous transmission. Instead, it waits a random period of time and then repeats the algorithm. Consequently, this algorithm leads to better channel utilization but longer delays than 1-persistent CSMA.
3. P-persistent CSMA:
The last protocol is p-persistent CSMA. It applies to slotted channels and works as follows. When a station becomes ready to send, it senses the channel. If it is idle, it transmits with a probability p. With a probability q = 1 - p, it defers until the next slot. If that slot is also idle, It either transmits or defers again, with probabilities p and q. This process is repeated until either the frame has been transmitted or another station has begun transmitting. In the latter case, the unlucky station acts as if there had been a collision (i.e., it waits a random time and starts again). If the station initially senses the channel busy, it waits until the next slot and applies the above algorithm. Figure 4 shows the computed throughput versus offered traffic for all three protocols, as well as for pure and slotted ALOHA.
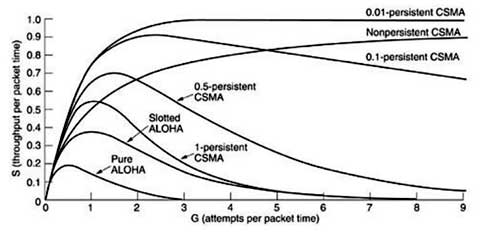
Fig.4 Comparison of the channel utilization versus load for various random access protocols
4.1.3 CSMA with Collision Detection:
Persistent and nonpersistent CSMA protocols are clearly an improvement over ALOHA because they ensure that no station begins to transmit when it senses the channel busy. Another improvement is for stations to abort their transmissions as soon as they detect a collision. In other words, if two stations sense the channel to be idle and begin transmitting simultaneously, they will both detect the collision almost immediately. Rather than finish transmitting their frames, which are irretrievably garbled anyway, they should abruptly stop transmitting as soon as the collision is detected. Quickly terminating damaged frames saves time and bandwidth.
This protocol, known as CSMA/CD (CSMA with Collision Detection) is widely used on LANs in the MAC sub layer. In particular, it is the basis of the popular Ethernet LAN, so it is worth devoting some time to looking at it in detail. CSMA/CD, as well as many other LAN protocols, uses the conceptual model of Fig.4. At the point marked t0, a station has finished transmitting its frame. Any other station having a frame to send may now attempt to do so. If two or more stations decide to transmit simultaneously, there will be a collision. Collisions can be detected by looking at the power or pulse width of the received signal and comparing it to the transmitted signal.
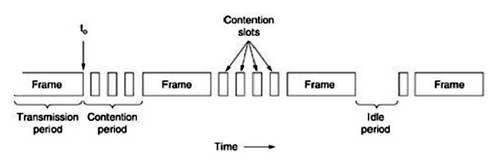
Fig.4. CSMA/CD can be in one of three states: contention, transmission, oridle
After a station detects a collision, it aborts its transmission, waits a random period of time, and then tries again, assuming that no other station has started transmitting in the meantime. Therefore, our model for CSMA/CD will consist of alternating contention and transmission periods, with idle periods occurring when all stations are quiet (e.g., for lack of work).
Now let us look closely at the details of the contention algorithm. Suppose that two stations both begin transmitting at exactly time t0. How long will it take them to realize that there has been a collision? The answer to this question is vital to determining the length of the contention period and hence what the delay and throughput will be. The minimum time to detect the collision is then just the time it takes the signal to propagate from one station to the other.
Based on this reasoning, you might think that a station not hearing a collision for a time equal to the full cable propagation time after starting its transmission could be sure it had seized the cable. By ''seized,'' we mean that all other stations knew it was transmitting and would not interfere. This conclusion is wrong. Consider the following worst-case scenario. Let the time for a signal to propagate between the two farthest stations be. At t0, one station begins transmitting. At an instant before the signal arrives at the most distant station, that station also begins transmitting. Of course, it detects the collision almost instantly and stops, but the little noise burst caused by the collision does not get back to the original station until time. In other words, in the worst case a station cannot be sure that it has seized the channel until it has transmitted for without hearing a collision. For this reason we will model the contention interval as a slotted ALOHA system with slot width.
Published date : 18 Jul 2015 03:50PM