Citiric acid Cycle
Sakshi Education
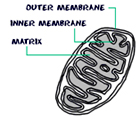
Cellular respiration a process where the cells consume O2 and generate CO2
Respiration is divided into three phases:
In the first phase, organic compounds (Carbohydrates, Amino acids and Fatty acids) are oxidized to two carbon compound, acetyl-CoA.
In the second phase, acetyl-CoA is further oxidized to CO2 and releases the NADH and FADH2.
In the third phase, ATP is generated by the oxidation of NADH and FADH2 via electron transport chain.
Glycolysis (Phase 1) occurs in the cytosol where as in eukaryotes Citric acid Cycle, electon transport chain and oxidative phosphorylation occur in the mitochondrial matrix.
Citiric acid cycle is also known as Tricarboxlylic acid cycle of Krebs cycle. It is considered as the metabolic hub because several catabolic and anabolic pathways converge at this cycle.
In the first step pyruvate derived from glycolysis is converted to acetyl-CoA, the reaction is irreversible and is catalyzed by a multienzyme complex – Pyruvate dehydrogenase (PDH) complex.
PDH complex is made of 3 enzymes {pyruvate dehydrogenase (E1), dihydrolipoyl transacetylase (E2) and dihydrolipoyl dehydrogenase (E3)} and five coenzymes {Thiamine pyrophosphate (TPP), Flavin adenine dinucleotide (FAD) Nicotinamide adenine }
The overall reaction catalyzed during this step is as follows:
Pyruvate + CoA + NAD acetyl CoA + CO2 + NADH + H+
E1 catalyzes the decarboxylation of pyruvate.
E2 catalyzes the transfer of acetyl group to coenzyme-A.
E3 catalyzes the regeneration of oxidized lipoate and production of NADPH and FADH2.
Acetyl CoA formed in the reaction catalyzed by PDH complex enters into the Citric acid cycle, where in series of 8 reactions – one molecule of acetyl CoA is converted into two molecules CO2 and the energy released is conserved in the form in the form of NADH and FADH2
The overall reaction of citric acid cycle is as follows:
Acetyl CoA + 3NAD+ + FAD + GDP + Pi +2H2O 2CO2 + 3NADH + FADH2 + GTP + 2H+ + CoA
Reaction 1: Formation of Citrate
The first step in the cycle is the condensation of 2 carbon acetyl-CoA with the four carbon oxaloacetate to form citrate catalyzed by the enzyme Citrate synthase. This reaction is aldol condensation followed by hydrolysis which involves an intermediate in the form of citroyl CoA and this is the first committed step of the cycle.
Reaction 2: Isomerization
Decarboxylation usually occurs on a-keto acids or a-hydroxy acids. As citrate is not a good substrate for decarboxylation, in this reaction citrate by the removal and addition of a water molecule is converted to isocitrate (a-hydroxy acid) by the enzyme aconitase. Aconitase is a iron-sulfur protein, this iron-sulfur is involved in removal and addition of water. The equilibrium of the reaction is towards the reactants but the product is consumed readily so the reaction moves in a forward direction. Flouroacetate is non-competitive inhibitor.
Reaction 3: Oxidative decarboxylation
This is first of the two oxidative decarboxylation reactions present in citric acid cycle. In this step Isocitrate dehydrogenase catalyzes the removal of CO2 to form a-ketoglutarate. Oxalosuccinate, a ß-keto acid is formed as an intermediate in the reaction.
Isocitrate dehydrogenase is present in two isoforms one requires NAD+ and the other NADP as elector acceptor. NAD+ dependent is present in matrix where as NADP+ present in both matrix and cytosol and is involved in anabolic reactions.
Reaction 4: Oxidative decarboxylation
In the second oxidative decarboxylation reaction catalyzed by a-ketoglutarate dehydrogensase involves the removal of CO2 and addition of CoA to form Succinyl CoA. NAD+ acts as the electron acceptor and CoA as the carrier of succinyl group. This reaction is similar to the reaction catalyzed by pyruvate dehydrogenase complex. Arsinite is a non-competitive inhibitor.
The next four cycles serves to regenerate substrate oxaloacetate.
Reaction 5: Substrate level phosphorylation
Succinyl CoA formed in the above reaction has astrong negative standard free energy of hydrolysis. In this reation succinyl CoA is hydrolysed by the enzyme Succinyl CoA synthetase to form Succinate and GTP. This enzyme has two subunits a and ß, a-subunit has the binding site for CoA and ß-subunit has the binding site for either GDP or ADP
GTP is converted to ATP by the enzyme Nucleoside diphosphokinase
In the case of plants ATP is directly formed.
Reaction 6: Oxidation:
Succinate is oxixdized to fumarate by the enzyme Succinate dehydrogenase. In this reaction C-C bond is oxidized to C=C bond. FAD acts as the coenzyme where it accepts the hydrogen and forms FADH2. In eukaryotes Succinate dehydrogenase is bound to the inner membrane of the mitochondria, where as in prokaryotes to the plasma membrane. This enzyme contains iron-sulfur clusters and covalently bound FAD, electrons transfer from these clusters to FAD before they enter into electron transport chain.
Malonate, analogue of succinate acts as a competitive inhibitor of succinate dehydrogenase
Reaction 7: Hydration
Water molecule is reversibly added to the double bond of fumarate to form Malate catalyzed by Fumarase. Fumarase is a stereospecific it adds water molecule only to the trans double bond of fumarate.
Reaction 8: Oxidation
In the last reaction Malate dehydrogenase catalyzes the oxidation of malate to regenerate oxaloacetate. NAD+ acts the hydrogen acceptor. The equilibrium of the reaction lies towards the substrate, as the oxaloacetate formed is readily used by the enzyme citrate synthase the reaction so the reation moves towards the formation products (Oxaloacetate).
Overall reaction of Citric acid cycle:
Acetyl CoA + 3 NAD+ + FAD + GDP +Pi + 2 H2O 2 CO2 + 3 NADH + FADH2 + CoASH + GTP + 3 H+
One turn of Citric acid cycle generates 3 NADH, one FADH and one GTP (which later on converts into ATP)
Regulation of Citric acid Cycle:
Citric acid cycle besides being important for generating cellular energy it is also the source for generating biosynthetic intermediates.
This cycle is regulated by three simple steps:
Respiration is divided into three phases:
In the first phase, organic compounds (Carbohydrates, Amino acids and Fatty acids) are oxidized to two carbon compound, acetyl-CoA.
In the second phase, acetyl-CoA is further oxidized to CO2 and releases the NADH and FADH2.
In the third phase, ATP is generated by the oxidation of NADH and FADH2 via electron transport chain.
Glycolysis (Phase 1) occurs in the cytosol where as in eukaryotes Citric acid Cycle, electon transport chain and oxidative phosphorylation occur in the mitochondrial matrix.
Citiric acid cycle is also known as Tricarboxlylic acid cycle of Krebs cycle. It is considered as the metabolic hub because several catabolic and anabolic pathways converge at this cycle.
In the first step pyruvate derived from glycolysis is converted to acetyl-CoA, the reaction is irreversible and is catalyzed by a multienzyme complex – Pyruvate dehydrogenase (PDH) complex.
PDH complex is made of 3 enzymes {pyruvate dehydrogenase (E1), dihydrolipoyl transacetylase (E2) and dihydrolipoyl dehydrogenase (E3)} and five coenzymes {Thiamine pyrophosphate (TPP), Flavin adenine dinucleotide (FAD) Nicotinamide adenine }
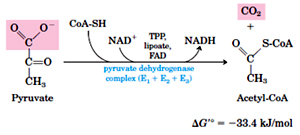
Pyruvate + CoA + NAD acetyl CoA + CO2 + NADH + H+
E1 catalyzes the decarboxylation of pyruvate.
E2 catalyzes the transfer of acetyl group to coenzyme-A.
E3 catalyzes the regeneration of oxidized lipoate and production of NADPH and FADH2.
Acetyl CoA formed in the reaction catalyzed by PDH complex enters into the Citric acid cycle, where in series of 8 reactions – one molecule of acetyl CoA is converted into two molecules CO2 and the energy released is conserved in the form in the form of NADH and FADH2
The overall reaction of citric acid cycle is as follows:
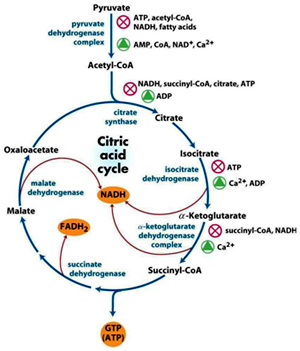
Reaction 1: Formation of Citrate

Reaction 2: Isomerization
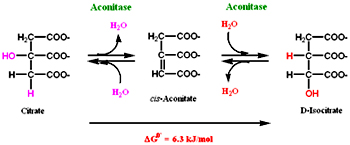
Reaction 3: Oxidative decarboxylation
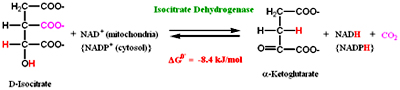
Isocitrate dehydrogenase is present in two isoforms one requires NAD+ and the other NADP as elector acceptor. NAD+ dependent is present in matrix where as NADP+ present in both matrix and cytosol and is involved in anabolic reactions.
Reaction 4: Oxidative decarboxylation
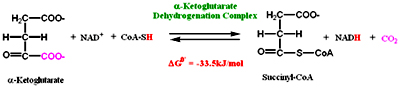
The next four cycles serves to regenerate substrate oxaloacetate.
Reaction 5: Substrate level phosphorylation
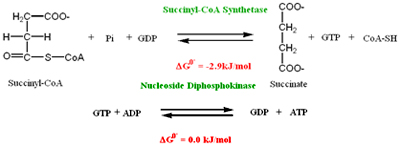
GTP is converted to ATP by the enzyme Nucleoside diphosphokinase
In the case of plants ATP is directly formed.
Reaction 6: Oxidation:
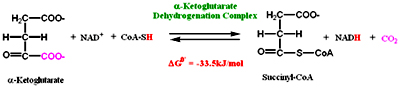
Malonate, analogue of succinate acts as a competitive inhibitor of succinate dehydrogenase
Reaction 7: Hydration
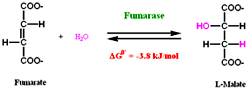
Reaction 8: Oxidation
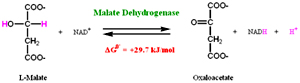
Overall reaction of Citric acid cycle:
Acetyl CoA + 3 NAD+ + FAD + GDP +Pi + 2 H2O 2 CO2 + 3 NADH + FADH2 + CoASH + GTP + 3 H+
One turn of Citric acid cycle generates 3 NADH, one FADH and one GTP (which later on converts into ATP)
Regulation of Citric acid Cycle:
Citric acid cycle besides being important for generating cellular energy it is also the source for generating biosynthetic intermediates.
This cycle is regulated by three simple steps:
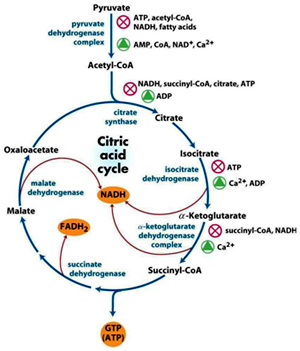
- Feedback mechanism
- Availability of substrate
- Product Inhibition
- Pyruvate dehydrogenase reaction
- Citrate synthase reaction
- Isocitrate dehydrogenase reaction
- a-keto glutarate dehydrogenase reaction
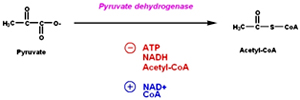
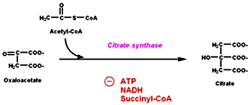
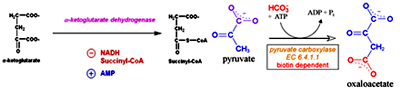
Anaplerotic Reactions:
Anaplerotic reactions are those that form the intermediates of the metabolic reaction. Intermediates of citric acid cycles serves as biosynthetic precursors, these intermediates have to be refilled. The reactions involved in refilling the intermediates are called anaplerotic reactions.
- Carboxylation of pyruvate to oxaloacetate: When the levels of oxaloacetate are low, pyruvate carboxylase (PC) catalyzes the addition of CO2 to pyruvate to form oxaloacetate, this is the major anaplerotic enzymes in the cell. This enzymatic addition requires ATP and Mg. This reaction takes place in mitochondria. Pyruvate carboxylase is activated by acetyl-CoA and inhibited by high concentrations of many acyl-CoA derivatives. As the concentration of oxaloacetate is depleted through the efflux of TCA cycle intermediates, the rate of the citrate synthase reaction decreases and acetyl-CoA concentration rises. The acetyl-CoA then activates pyruvate carboxylase to synthesize more oxaloacetate. PC is a biotin dependent enzyme. Biotin, a single carbon carrier plays a key role in carboxylation reactions. Carboxyl groups are activated in a step where ATP is utilized and joins CO2 to biotin. This activated CO2 id then joined to acceptor (pyruvate).
- Transamination of aspartate to oxaloacetate: The reversible transamination of the a-amino group from L-aspartate to a-ketoglutarate forming oxaloacetate and glutamate. This reaction is catalyzed by amino-transferases or transaminases, in this case specifically by aspartate amino-transferase and this enzyme depends on the cofactor pyridoxal phosphate (PLP). PLP functions as an intermediate carrier of amino groups at the active site of aminotransferases. When amino acid is added, a Schiff base will form between the enzyme and PLP which causes the aminoacid to lose a hydrogen form quinoid intermediate, and reprotanation forms ketimine. Then the structure is hydrolyzed forming an a-keto acid and pyridoxamine phosphate. 2-methyl aspartate acts as inhibitor of aspartate aminotransferase.
- Hydration of glutamate to a-ketoglutarate: In this reaction glutamate undergoes oxidative deamination to form ammonia and a-ketoglutarate, catalyzed by glutamate dehydrogenase. This enzyme can use either NAD+ or NADP+ as coenzyme.
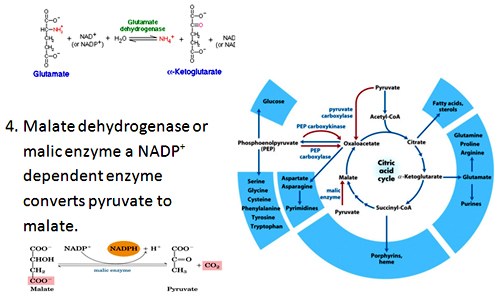
Oxaloacetate and a-ketoglutatrate serves as precursor’s for amino acids aspartate and glutamate by transamination. These are also involved in purine and pyrimidine synthesis Oxaloacetate is converted to glucose by gluconeogenesis.
Succinyl-CoA is used in the synthesis of porphyrin ring of heme groups.
ELECTRON TRANSPORT CHAIN and OXIDATIVE PHOSPHORYLATION:
In aerobic organisms Electron transport chain and Oxidative phosphorylation is the last phase of cellular respiration. All the catabolic pathways (carbohydrate, amino acid, fatty acid) intersect at this stage, where the energy of oxidation drives the synthesis of ATP.
In eukaryotes these reactions takes place in Mitochondria where as in prokaryotes as they lack the cell organelles, electron transport chain and oxidative phosphorylation occurs across the plasma membrane.
Mitochondria:
Mitochondrion, a membrane bound organelle found in the cytoplasm of all eukaryotic cells, the main function of it is to generate energy in the form of ATP, so called as the power house of the cell. Besides it role in generation of ATP it is also involved in metabolism of amino acids and lipids, storage of calcium, cell signaling, generation of heat, cell growth, cell differentiation and death. Mitochondria are typically round to oval in shape ranging in size from 0.5 to 10µm. Unlike other organelles mitochondria is surrounded by a double membrane, have its own genome and reproduce independent of cell division by binary fission, their number per cell varies widely. Due to their independence from the nuclear DNA and similarities with bacteria, it is believed that mitochondria have originated from bacteria by endosymbiosis.
The mitochondrial shape also varies depending on the cell type, from the tubular-shaped mitochondria in many cell types to mitochondria that consist of sheets and have a spherical-shape structures that are enclosed within two membranes - the outer membrane and the inner membrane. The membranes are made up of phospholipids and proteins. The structures of the various components of mitochondria are as follows:
· Outer membrane
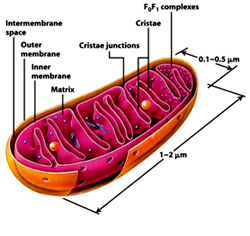
• Inter membrane space
The space in between outer and inner mitochondrial membrane is called as intermembrane space or perimitochondrial space. The concentration of ions and sugars in intermembrane space is same as that of cytosol due to the freely permeable nature of outer membrane. However the protein differs from that of cytosol. Cytochrome c is localized to this space.
• Inner Membrane
The inner membrane is more complex in structure than the outer membrane as it contains the complexes of the electron transport chain and the ATP synthetase complex. It is permeable only to oxygen, carbon dioxide and water. It is made up of a large number of proteins that play an important role in producing ATP, and also helps in regulating transfer of metabolites across the membrane. The inner membrane has infoldings called the cristae that increase the surface area for the complexes and proteins that aid in the production of ATP, the energy rich molecules. Inner membrane is rich in phospholipid, Cardiolipin-which makes the membrane impermeable. The TIM Complex (translocase of inner membrane) translocates the ions and molecules across the inner membrane.
• Matrix
The matrix is a complex mixture of enzymes that are important for the synthesis of ATP molecules, special mitochondrial ribosome’s, tRNAs and the mitochondrial DNA. Besides these, it has oxygen, carbon dioxide and other recyclable intermediates. Major functions are the oxidation of pyruvate, fatty acids and amino acids.
Human mitochondrial DNA is of 16,569 base pairs encoding 37 total genes: 22 tRNA, 2 rRNA, and 13 peptide genes. These 13 peptides are integrated into the inner mitochondrial membrane along with proteins encoded by genes that are present on host cell’s nucleus. Most of them are encoded by nuclear genome which are synthesized on cytosolic ribosomes and then imported into different compartments of mitochondria using protein specific import mechanisms.
Steps of protein import into mitochondria General import pathway [Pfanner et.al 1990]:
- Synthesis of proteins on cytosolic ribosome’s as pre-proteins and release into the cytosol.
- Transfer to the mitochondria assisted by cytosolic factors that help to maintain import competence and prevent aggregation.
- Recognition of pre-proteins by interaction of mitochondrial targeting signals with receptors on the surface of the outer membrane of mitochondria
- Initiation of transfer through the pre-protein translocase complex of the outer membrane (TOM complex)
- Interaction of pre-proteins with the surface of the inner membrane and insertion into translocase of the inner membrane (TIM complex) triggered by the membrane potential
- Completion of translocation through outer and inner membrane by the matrix-localized mt-Hsp70-ATP–dependent driving system associated with the TIM complex
- Proteolytic processing of pre-proteins with cleavable targeting signals in the matrix
- Folding of proteins in the matrix assisted by the molecular chaperone systems, mt-Hsp70, Hsp60, and associated co-chaperone.
- Pre-sequences direct precursor proteins via the TOM complex to the TIM23 translocase, where they are sorted to the matrix, inner membrane or intermembrane space. These presequences are of 20-30 amino acids residues capable of folding into a positively charged amphiphilic helix which is important for receptor recognition.
- In comparison, proteins which only contain internal targeting signals are directed to either the inner membrane, intermembrane space or outer membrane, depending on the nature of the signal (Baker MJ et.al 2007).
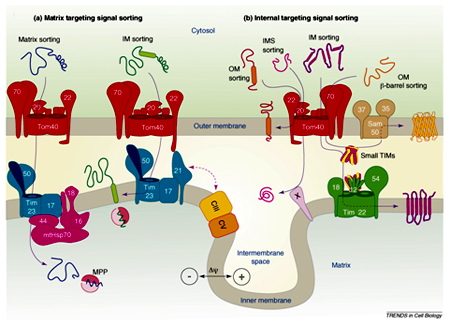
Published date : 31 May 2014 04:25PM